ABSTRACT
Objective: To determine the role of the IL8 rs4073 polymorphism in predicting the risk of central nervous system (CNS) toxicity in patients receiving standard pharmacological treatment for multidrug-resistant tuberculosis (MDR-TB). Methods: A cohort of 85 consenting MDR-TB patients receiving treatment with second-line antituberculosis drugs had their blood samples amplified for the IL8 (rs4073) gene and genotyped. All patients were clinically screened for evidence of treatment toxicity and categorized accordingly. Crude and adjusted associations were assessed. Results: The chief complaints fell into the following categories: CNS toxicity; gastrointestinal toxicity; skin toxicity; and eye and ear toxicities. Symptoms of gastrointestinal toxicity were reported by 59% of the patients, and symptoms of CNS toxicity were reported by 42.7%. With regard to the genotypes of IL8 (rs4073), the following were identified: AA, in 64 of the study participants; AT, in 7; and TT, in 11. A significant association was found between the dominant model of inheritance and CNS toxicity for the crude model (p = 0.024; OR = 3.57; 95% CI, 1.18-10.76) and the adjusted model (p = 0.031; OR = 3.92; 95% CI, 1.13-13.58). The AT+TT genotype of IL8 (rs4073) showed a 3.92 times increased risk of CNS toxicity when compared with the AA genotype. Conclusions: The AT+TT genotype has a tendency to be associated with an increased risk of adverse clinical features during MDR-TB treatment.
Keywords:
Tuberculosis, multidrug-resistant; Immunity; Pharmacogenetics; Polymerase chain reaction; Risk.
RESUMO
Objetivo: Determinar o papel do polimorfismo rs4073 do gene IL8 na previsão do risco de toxicidade do sistema nervoso central (SNC) em pacientes em tratamento farmacológico padrão para tuberculose multirresistente (TBMR). Métodos: Amostras de sangue de uma coorte de 85 pacientes com TBMR que assinaram um termo de consentimento livre e esclarecido e que estavam recebendo tratamento com medicamentos antituberculosos de segunda linha foram amplificadas para o gene IL8 (rs4073) e genotipadas. Todos os pacientes foram avaliados clinicamente quanto a evidências de toxicidade do tratamento e categorizados de acordo com os achados. Foram avaliadas as associações brutas e ajustadas. Resultados: As principais queixas enquadraram-se nas seguintes categorias: toxicidade do SNC; toxicidade gastrointestinal; toxicidade cutânea; e toxicidade ocular e ototoxicidade. Sintomas de toxicidade gastrointestinal foram relatados por 59% dos pacientes, e sintomas de toxicidade do SNC foram relatados por 42,7%. Foram identificados os seguintes genótipos de IL8 (rs4073): AA, em 64 dos participantes; AT, em 7; TT, em 11. Houve associação significativa entre o modelo dominante de herança e toxicidade do SNC no modelo bruto (p = 0,024; OR = 3,57; IC95%: 1,18-10,76) e no ajustado (p = 0,031; OR = 3,92; IC95%: 1,13-13,58). O genótipo AT+TT do gene IL8 (rs4073) apresentou risco 3,92 vezes maior de toxicidade do SNC que o genótipo AA. Conclusões: O genótipo AT+TT tende a se associar a um maior risco de características clínicas adversas durante o tratamento da TBMR.
Palavras-chave:
Tuberculose resistente a múltiplos medicamentos; Imunidade; Farmacogenética; Reação em cadeia da polimerase; Risco.
INTRODUCTION Tuberculosis disease is very common in poor countries with a high population of HIV-infected individuals, malnourished individuals, or both. Nigeria is one of the countries with the highest tuberculosis burden, with a prevalence of 830,000 cases.(1,2) A poor tuberculosis infection control program is characterized by low (below-expected) rates of disease diagnosis and insufficient or inadequate treatment of diagnosed tuberculosis, leading to treatment failure,(1) which can be attributed to poor treatment compliance or a lack of effective drugs available in the national health care systems.(3) Treatment failure is most pronounced in patients diagnosed with multidrug-resistant tuberculosis (MDR-TB). Approximately 45% of all treated cases reportedly fail to achieve a successful outcome, and this category of treatment outcome has an enormous role in the continued persistence of tuberculosis disease.(4) Another very important factor contributing to poor treatment compliance and treatment failure is the adverse drug reaction (ADR) commonly associated with tuberculosis treatment. It is of note that there are numerous potential ADRs, such as hepatotoxicity, cardiotoxicity, and nephrotoxicity, which could actually be detected by tests incorporated into a holistic tuberculosis management protocol. Routine liver enzyme testing, assessment of renal function biomarkers, and echocardiography can be incorporated into the tuberculosis management protocol in order to allow early detection. A sizable number of patients express frustration with their antituberculosis medications and stop taking them well before any objective findings suggesting toxicity.(5) Thus, identifying clinical symptoms that may herald the manifestation of a devastating ADR may provide an early predictor of the ADR.
Neurotoxicity, which can be central or peripheral, initially manifests via a number of very wide-ranging clinical features, including headache, blurred vision, tremors, dizziness, tinnitus, and poor coordination. (6-9) Other severe and highly morbid manifestations of neurotoxicity include seizure, loss of vision, ataxia, psychosis, myoclonus, and delirium.
Increased perspiration, rashes, dry skin, and itching are common early features of skin-related antituberculosis drug toxicity, whereas Stevens-Johnson syndrome is a life-threatening feature.(10) Antituberculosis drugs can cause reversible blurred vision, and permanent visual impairment can occur in severe cases.(11,12)
There is a clear difference among patients regarding the occurrence of ADRs. Some of the risk factors for ADRs are well known, whereas others have yet to be identified.(13) In addition to establishing an accurate diagnosis of tuberculosis, identifying risk factors for individual differences in ADRs in patients receiving tuberculosis treatment is very important to avoid iatrogenic drug injury in susceptible patients.
Polymorphisms in drug-metabolizing genes such as NAT2, GSTM1, GSTT1, and CYP2E1 have been implicated in the considerably complex variability of antituberculosis drug levels and their effects, including ADRs.(13,14) It is well known that environmental factors can modify the effects of these pharmacogenetic variations on the metabolism of antituberculosis drugs.(13) A number of pharmacogenetic studies have investigated the role of genetic polymorphisms of proinflammatory cytokines such as TNF, IL-6, and IL-8 in first-line tuberculosis treatment outcomes such as ADRs.(14-16) It is also well established that the human genome has a number of common genetic variants that are in linkage disequilibrium and that are inheritable, being inherited in unison along with all of the yet to be identified genetic variants responsible for the clinical phenotypes (disease/treatment outcome—efficacy or ADR).(17) Thus, the well-established drug-metabolizing enzyme gene loci associated with antituberculosis drugs might be in linkage with the gene loci of proinflammatory cytokines.(17-19) The impetus for the present study was the dearth of pharmacogenomic studies assessing ADRs to second-line antituberculosis drugs, which are more toxic by nature, especially with regard to neurotoxicity, which is not monitored by periodic serological testing in the management of tuberculosis. In addition, the current WHO global action framework for tuberculosis research encourages patient-oriented tuberculosis research and innovations at country level, especially in low- and middle-income countries.(3,18)
METHODS Study location and design The study design was approved by the Kano State Ministry of Health Research Ethics Committee (NHREC/17/03/2018). Tuberculosis patients were recruited among those receiving hospital-based MDR-TB treatment and those receiving MDR-TB treatment in a community care setting in Kano, Nigeria. The study assessed associations of single nucleotide polymorphisms with clinical phenotypes of toxicity following MDR-TB treatment with second-line antituberculosis drugs. Clinical toxicities were assessed by the Patient-Rated Inventory of Side Effects and included gastrointestinal symptoms such as nausea, vomiting, constipation, diarrhea, abdominal pain, and loss of appetite, as well as central nervous system (CNS) symptoms such as dizziness, drowsiness, headache, poor coordination, restlessness, and tremors.
A cohort of 85 patients diagnosed with and receiving treatment for MDR-TB were enrolled in this study. The MDR-TB treatment regimen used in our clinic included levofloxacin, bedaquiline, ethionamide, cycloserine, delamanid, pyrazinamide, meropenem, linezolid, and moxifloxacin. Patients received the WHO-recommended doses, always in accordance with weight, BMI, or both.(20) All patients were diagnosed at a tuberculosis center by a trained physician using WHO-recommended diagnostic criteria. MDR-TB was defined as a positive Xpert MTB/RIF assay result (Cepheid, Sunnyvale, CA, USA). The inclusion criteria were as follows: laboratory and/or genetic evidence of MDR-TB at the onset of therapy; evidence of optimal adherence to and completion of treatment with second-line antituberculosis drugs; having no immunodeficiency or other diseases; and being in the 18- to 80-year age bracket. The exclusion criteria were as follows: being very ill and incapable of providing written informed consent; having no documented evidence of an MDR-TB diagnosis; currently receiving treatment with first-line antituberculosis drugs; having no documented evidence of compliance with treatment; being pregnant; having hepatitis; and having declined to give written informed consent.
Five milliliters of peripheral venous blood were collected from consenting participants after completion of treatment, with the use of a 5-mL syringe fitted with an appropriate sized 18-G needle. The blood was anticoagulated with 0.5% EDTA (pH = 8.0) by placing it in an EDTA tube. Genomic DNA was extracted by using a whole blood genomic DNA extraction kit (QIAGEN, Hilden, Germany) in accordance with the manufacturer instructions, with an appropriate quantity of molecular-grade ethanol (Sigma-Aldrich, Burlington, MA, USA), being stored at −20°C for later use.
The genotypes of IL8 (rs4073) were amplified by using the appropriate identified forward primer: 5′-ATCTTGTTCTAACACCTGCCACTC-3′ and reverse primer 5′-TAAAATACTGAAGCTCCACAATTTGG-3′ in the reaction mixture. The reaction mixture was made up to a total volume of 25 µL containing 12.5 µL of DreamTaq Green PCR Master Mix (Thermo Fisher Scientific, Waltham, MA, USA), 5 µL of template DNA, 1 µL of upstream primer, 1 µL of downstream primer, and 5.5 µL of RNase-free water. The PCR cycling conditions consisted of an initial denaturation step at 94°C for 5 min, followed by 35 cycles at 94°C for 50 s, 61°C for 60 s, and 72°C for 55 s, followed by a final extension step at 72°C for 5 min. The PCR product was digested with the MfeI restriction enzyme (Thermo Fisher Scientific) at 37°C for 2 h and deactivated at 80°C for 20 min. The digested PCR product was analyzed by 2% agarose gel electrophoresis, set up in ultrapure grade Tris-acetate-EDTA buffer solution, visualized under ultraviolet illumination, scanned, and photographed.(21)
Sample size calculation For the current study, the sample size was calculated for a power of 80% and a level of significance of 5% to identify an OR difference of at least two-fold (OR = 2) in the distribution of genotypes for MDR-TB, which has a prevalence of 32% in Nigeria.(22) The estimates from the literature were substituted into the Schlesselman formula for sample size calculation:
M = m/Pe
where m = (((Z1-a/2/2 + Z1-b) (P*(1-P*))1/2)2 / (P*-0.5)2; P* = OR/(1 + OR); Pe (probability of exposure-discordant pair) = (p1(1-p0) + p0(1-p1); p1(proportion of exposed individuals developing case) = p0 (OR)/1 + p0 (OR-1); and p0 = exposure rate among controls in populations.
The estimated sample size for cases and controls was 30 each, for a total of 60 (m = 14.495; Pe = 0.118; P* = 0.667; p1 = 0.485; M = 30).
RESULTS The sociodemographic characteristics of the study participants are described in Table 1. The study participants were mostly poor (n = 74; 87.06%), even though they earned some income through their jobs (n = 77; 90.59%). The mean age of the participants was 31.88 ± 11.51 years. Most were of the Hausa ethnic group, were Muslims (Islam), were employed, and were from low-income families (Table 1).
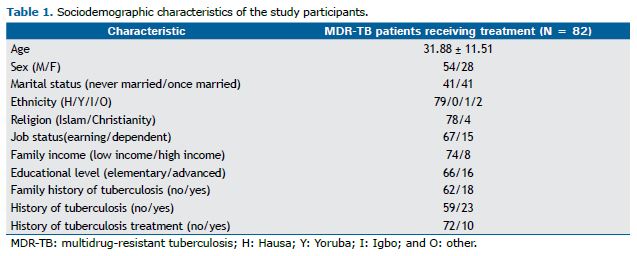
With regard to the clinical characteristics of the study participants, more than 22.5% had a history of tuberculosis/MDR-TB, a history of tuberculosis treatment, a family history of tuberculosis, or any combination of the three. As can be seen in Table 2, the study participants reported the following MDR-TB treatment-related symptoms: gastrointestinal symptoms, sleep symptoms, CNS symptoms, skin symptoms, cardiovascular symptoms, and urinary tract symptoms. CNS symptoms included the following: headache (in 9.8%), tremors (in 14.6%), poor coordination (in 2.4%), and dizziness (in 11%). Gastrointestinal symptoms included the following: vomiting/nausea (in 30.1%), diarrhea (in 7.2%), and constipation (in 2.4%). Blurred vision and ringing in the ears (tinnitus) were also reported (by 7.2% and 13.3%, respectively).
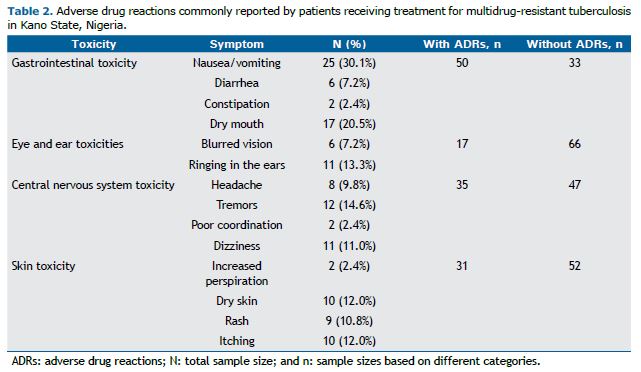
With regard to the genotypes of IL8 (rs4073), the following were identified: AA (wild-type homozygous), in 64 of the study participants; AT (wild-type heterozygous), in 7; and TT (homozygous), in 11 (Table 3). None of the genotypes of IL8 (rs4073) were significantly associated with ADR phenotypes, the exception being the phenotype of CNS toxicity. Table 3 shows the distribution of the genotypes of IL8 (rs4073) by inheritance model and CNS toxicity phenotype.
A significant association was found between the dominant model of inheritance and the binary dependent variable (presence or absence of ADRs involving the CNS) for the crude model (p = 0.024; OR = 3.57; 95% CI, 1.18-10.76) and the adjusted model (p = 0.031; OR = 3.92; 95% CI, 1.13-13.58; Table 3). The AT+TT genotype of IL8 (rs4073) showed a 3.92 times increased risk of CNS toxicity when compared with the AA genotype.
DISCUSSION In our sample of patients with MDR-TB, there were more males than females (n = 58 vs. n = 28), and the numbers of married and unmarried participants were the same (n = 41 for both). The religious and ethnic characteristics of the study participants were consistent with those predominantly seen in the population of Kano State, Nigeria. The predominance of individuals with low income and an elementary level of education in our sample of patients with MDR-TB was similar to what is observed in other areas where tuberculosis is endemic. A significant association was found between the dominant model of inheritance of IL8 (rs4073) genotypes and CNS toxicity. The presence of the T allele (in the AT+TT genotype) significantly increased the risk of CNS toxicity (by 3.92 times).
To the best of our knowledge, our finding of an association between genotypes of IL8 (rs4073) and CNS toxicity is a novel result. Although earlier studies have assessed the association of IL8 rs4073 with phenotypes of tuberculosis disease, there have been no studies assessing the association of IL8 (rs4073) with phenotypes of treatment-related ADRs. There have been reports of an association between IL8 (rs4073) and other medical conditions, including breast cancer and autoimmune thyroid disease.(23,24) In the aforementioned studies,(23,24) the TT genotype was associated with a higher risk of breast cancer or autoimmune thyroid disease than was the AA genotype. In another study,(25) the IL8 (rs4073) polymorphism was assessed in its dominant, recessive, and allele models, and was associated with a risk of developing pulmonary tuberculosis disease. Thus, there is a similarity in the role of the TT genotype between our study and other studies in the literature regarding its tendency to be associated with an increased risk of adverse clinical features, despite the difference in the clinical features assessed; the aforementioned studies assessed breast cancer, tuberculosis, and autoimmune thyroid disease, whereas our study assessed symptoms of CNS toxicity. The precise mechanism by which this polymorphism influences the development of diseases such as breast cancer, tuberculosis, and autoimmune thyroid disease has yet to be elucidated. Understandably, the role of the genotypes in the development of toxicities of second-line antituberculosis drugs has yet to be established as well. However, the mechanism by which cytokines contribute to toxicity in any organ might be similar to the mechanisms of hepatotoxicity. Hepatotoxicity is the most studied toxicity mechanism for antituberculosis drugs, and the identified mechanisms of hepatotoxicity might be similar to those of other toxicities. It develops directly from the drug metabolites or indirectly via immune mediation.(26) Thus, the delicate balance between proinflammatory and anti-inflammatory cytokines plays a vital role in the progression of immune-mediated tissue injury. The anti-inflammatory mechanisms in the liver suppress the activities and production of proinflammatory factors such as TNF-α, IFN-c, IL-1, and IL-8, thus limiting the progression of hepatotoxicity.(27) In the same vein, the anti-inflammatory role of IL-8, which is abundantly secreted by neutrophils, antigen-presenting cells such as oligodendrocytes, and Schwann cells may putatively underlie its role in the development of toxicity in the CNS and other tissues. Furthermore, the activities of the proximal promoter region of the IL8 (rs4073) gene that modulates the transcriptional level of its proteins encoded by (four) exons interspersed within three intron regions may explain its role in the interindividual variability attributable to its different genotypes at the polymorphic site.(28) The aforementioned putative explanation appears very plausible and is in keeping with the finding of the current study, i.e., that the AT+TT genotype increases the risk of CNS toxicity. Nevertheless, a thorough mechanistic evaluation must be carried out for validation purposes.
One of the limitations of the current study was that we did not assess the association between the symptoms of CNS toxicity and the genotypes of the different drug-metabolizing enzymes. Future studies assessing the association of genotypes of drug-metabolizing enzymes and IL-8 (rs4073) with symptoms of toxicity could identify the presence or absence of linkage disequilibrium for these gene loci.
In conclusion, this study demonstrated an association between the IL8 rs4073 gene polymorphism and symptoms of CNS toxicity in MDR-TB patients receiving treatment with standard second-line drugs. This association must be confirmed by controlled studies.
ACKNOWLEDGMENTS The authors would like to thank the Kano State Ministry of Health and the Kano State Hospitals Management Board for allowing us to conduct this study. We would also like to thank the following people for their contribution to this study: Dr. Imam Wada Bello, Matron Hajia Zulai Sulaiman Tukur, Mal Aminu Tukur, Dr. Ibrahim Aliyu Umar, and Aishat Ahmad Umar at the Bayero University Kano Center for Infectious Diseases; and Dr. Ibrahim Sulaiman, Dr. Muktar Muhammad, and Mahmud Muhammad Rabiu. Finally, we would like to thank the Directorate of Research Innovation and Partnership for coordinating the funding.
AUTHOR CONTRIBUTIONS IBM, IAA, MAG, IAU, and JS developed the concept of the study. AUA, U-AMM, MM, OJ, I-MK, IBM, and IAU were involved in data collection. AUA, U-AMM, I-MK, and IBM were involved in genotyping. AUA, U-AMM, I-MK, IBM, JS, and MAG were involved in data analysis. All authors reviewed the manuscript.
CONFLICTS OF INTEREST None declared.
REFERENCES 1.Harshavardhan S, Vijayakumar KK, Sounderrajan V, Ramasamy P, Rajadas SE. Basics of tuberculosis disease and principles of treatment and their effects. In: Rajan M, editor. A Mechanistic Approach to Medicines for Tuberculosis Nanotherapy. London: Academic Press; 2021. p. 1-29. https://doi.org/10.1016/B978-0-12-819985-5.00011-5
2.Dinic L, Akande P, Idigbe EO, Ani A, Onwujekwe D, Agbaji O, et al. Genetic determinants of drug-resistant tuberculosis among HIV-infected patients in Nigeria. J Clin Microbiol. 2012;50(9):2905-2909. https://doi.org/10.1128/JCM.00982-12
3.Gonzalez Y, Guzmán-Beltrán S, Carreto-Binaghi LE, & Juárez E. Translational research for therapy against tuberculosis. In: Kesharwani P, editor. Nanotechnology Based Approaches for Tuberculosis Treatment. London: Academic Press; 2020. p. 53-73.doi:10.1016/b978-0-12-819811-7.00004-7 https://doi.org/10.1016/B978-0-12-819811-7.00004-7
4.World Health Organization. Global Health TB Report. Geneva: World Health Organization; 2018. ISBN 978-92-4-156564-6
5.Kaona FA, Tuba M, Siziya S, Sikaona L. An assessment of factors contributing to treatment adherence and knowledge of TB transmission among patients on TB treatment. BMC Public Health. 2004;4:68. https://doi.org/10.1186/1471-2458-4-68
6.Girling DJ. Adverse effects of antituberculosis drugs. Drugs. 1982;23(1-2):56-74. https://doi.org/10.2165/00003495-198223010-00003
7.Owens RC Jr, Ambrose PG. Antimicrobial safety: focus on fluoroquinolones. Clin Infect Dis. 2005;41 Suppl 2:S144-S157. https://doi.org/10.1086/428055
8.Schwartz MT, Calvert JF. Potential neurologic toxicity related to ciprofloxacin. DICP. 1990;24(2):138-140. https://doi.org/10.1177/106002809002400204
9.Narita M, Tsuji BT, Yu VL. Linezolid-associated peripheral and optic neuropathy, lactic acidosis, and serotonin syndrome. Pharmacotherapy. 2007;27(8):1189-1197. https://doi.org/10.1592/phco.27.8.1189
10.Kass JS, Shandera WX. Nervous system effects of antituberculosis therapy. CNS Drugs. 2010;24(8):655-667. https://doi.org/10.2165/11534340-000000000-00000
11.Blumberg HM, Burman WJ, Chaisson RE, Daley CL, Etkind SC, Friedman LN, et al. American Thoracic Society/Centers for Disease Control and Prevention/Infectious Diseases Society of America: treatment of tuberculosis. Am J Respir Crit Care Med. 2003;167(4):603-662. https://doi.org/10.1164/rccm.167.4.603
12.Menon V, Jain D, Saxena R, Sood R. Prospective evaluation of visual function for early detection of ethambutol toxicity. Br J Ophthalmol. 2009;93(9):1251-1254. https://doi.org/10.1136/bjo.2008.148502
13.Costa GN, Magno LA, Santana CV, Konstantinovas C, Saito ST, Machado M, et al. Genetic interaction between NAT2, GSTM1, GSTT1, CYP2E1, and environmental factors is associated with adverse reactions to anti-tuberculosis drugs. Mol Diagn Ther. 2012;16(4):241-250. https://doi.org/10.1007/BF03262213
14.Mattingly CJ, Rosenstein MC, Davis AP, Colby GT, Forrest JN Jr, Boyer JL. The comparative toxicogenomics database: a cross-species resource for building chemical-gene interaction networks. Toxicol Sci. 2006;92(2):587-595. https://doi.org/10.1093/toxsci/kfl008
15.García-Elorriaga G, Carrillo-Montes G, Mendoza-Aguilar M, González-Bonilla C. Polymorphisms in tumor necrosis factor and lymphotoxin A in tuberculosis without and with response to treatment. Inflammation. 2010;33(4):267-275. https://doi.org/10.1007/s10753-010-9181-8
16.Peresi E, Oliveira LR, da Silva WL, da Costa EA, Araujo JP Jr, Ayres JA, et al. Cytokine Polymorphisms, Their Influence and Levels in Brazilian Patients with Pulmonary Tuberculosis during Antituberculosis Treatment. Tuberc Res Treat. 2013;2013:285094. https://doi.org/10.1155/2013/285094
17.Motsinger AA, Ritchie MD. Multifactor dimensionality reduction: an analysis strategy for modelling and detecting gene-gene interactions in human genetics and pharmacogenomics studies. Hum Genomics. 2006 Mar;2(5):318-28. https://doi.org/10.1186/1479-7364-2-5-318
18.Piras D, Zoledziewska M, Cucca F, Pani A. Genome-Wide Analysis Studies and Chronic Kidney Disease. Kidney Dis (Basel). 2017;3(3):106-110. https://doi.org/10.1159/000481886
19.Deguchi T. Physiology and molecular biology of arylamine N-acetyl transferases. Biomed Res. 1992;13:231-242. https://doi.org/10.2220/biomedres.13.231
20.World Health Organization. Companion Handbook to the WHO Guidelines for the Programmatic Management of Drug- Resistant Tuberculosis. Geneva: World Health Organization; 2014. Available from: https://ncbi.nlm.nih.gov/books/NBK247420/?term=Companion%20Handbook%20to%20the%20WHO%20Guidelines%20for%20the%20Programmatic%20Management%20of%20Drug-%20Resistant%20Tuberculosis
21.Wu CC, Huang YK, Huang CY, Shiue HS, Pu YS, Su CT, et al. Polymorphisms of TNF-a -308 G/A and IL-8 -251 T/A Genes Associated with Urothelial Carcinoma: A Case-Control Study. Biomed Res Int. 2018;2018:3148137. https://doi.org/10.1155/2018/3148137
22.Onyedum CC, Alobu I, Ukwaja KN. Prevalence of drug-resistant tuberculosis in Nigeria: A systematic review and meta-analysis. PLoS One. 2017;12(7):e0180996. https://doi.org/10.1371/journal.pone.0180996
23.Zhang J, Han X, Sun S. IL-8 -251A/T and +781C/T polymorphisms were associated with risk of breast cancer in a Chinese population. Int J Clin Exp Pathol. 2017;10(7):7443-7450.
24.Akahane M, Watanabe M, Inoue N, Miyahara Y, Arakawa Y, Inoue Y, et al. Association of the polymorphisms of chemokine genes (IL8, RANTES, MIG, IP10, MCP1 and IL16) with the pathogenesis of autoimmune thyroid diseases. Autoimmunity. 2016;49(5):312-319. https://doi.org/10.3109/08916934.2015.1134507
25.Holt MP, Ju C. Mechanisms of drug-induced liver injury. AAPS J. 2006;8(1):E48-E54. https://doi.org/10.1208/aapsj080106
26.Bourdi M, Masubuchi Y, Reilly TP, Amouzadeh HR, Martin JL, George JW, et al. Protection against acetaminophen-induced liver injury and lethality by interleukin 10: role of inducible nitric oxide synthase. Hepatology. 2002;35(2):289-298. https://doi.org/10.1053/jhep.2002.30956
27.Chen J, Ma A. Associations of polymorphisms in interleukins with tuberculosis: Evidence from a meta-analysis. Immunol Lett. 2020;217:1-6. https://doi.org/10.1016/j.imlet.2019.10.012
28.Hacking D, Knight JC, Rockett K, Brown H, Frampton J, Kwiatkowski DP, et al. In-creased in vivo transcription of an IL-8 haplotype associated with respiratory syncytial virus disease-susceptibility. Genes Immun. 2004;5(4):274-282. https://doi.org/10.1038/sj.gene.6364067