ABSTRACT
Objective: To present a model of prostaglandin I2 (PGI2) administration (inhaled vs. parenteral) and to assess the functional performance of the lungs in an ex vivo lung perfusion system. Methods: Forty Wistar rats were anesthetized and placed on mechanical ventilation followed by median sterno-laparotomy and anticoagulation. The main pulmonary artery was cannulated. All animals were maintained on mechanical ventilation and were randomized into four groups (10 rats/group): inhaled saline (IS); parenteral saline (PS); inhaled PGI2 (IPGI2); and parenteral PGI2 (PPGI2). The dose of PGI2 used in the IPGI2 and PPGI2 groups was 20 and 10 µg/kg, respectively. The heart-lung blocks were submitted to antegrade perfusion with a low potassium and dextran solution via the pulmonary artery, followed by en bloc extraction and storage at 4°C for 6 h. The heart-lung blocks were then ventilated and perfused in an ex vivo lung perfusion system for 50 min. Respiratory mechanics, hemodynamics, and gas exchange were assessed. Results: Mean pulmonary artery pressure following nebulization decreased in all groups (p < 0.001), with no significant differences among the groups. During the ex vivo perfusion, respiratory mechanics did not differ among the groups, although relative oxygenation capacity decreased significantly in the IS and PS groups (p = 0.04), whereas mean pulmonary artery pressure increased significantly in the IS group. Conclusions: The experimental model of inhaled PGI2 administration during lung extraction is feasible and reliable. During reperfusion, hemodynamics and gas exchange trended toward better performance with the use of PGI2 than that with the use of saline.
Keywords:
Prostaglandins; Lung transplantation; Reperfusion; Models, animal; Rats.
RESUMO
Objetivo: Apresentar um modelo experimental de administração de prostaglandina I2 (PGI2) por via inalatória vs. parenteral e avaliar o desempenho funcional dos pulmões em um sistema de perfusão pulmonar ex vivo. Métodos: Quarenta ratos Wistar foram anestesiados, ventilados, submetidos a laparotomia com ressecção do esterno e anticoagulados. O tronco da artéria pulmonar foi canulado. Todos os animais foram submetidos a ventilação mecânica. Os animais foram randomizados em quatro grupos (10 ratos/grupo): salina nebulizada (SN); salina parenteral (SP); PGI2 nebulizada (PGI2N); e PGI2 parenteral (PGI2P). A dose de PGI2 nos grupos PGI2N e PGI2P foi de 20 e 10 µg/kg, respectivamente. Os blocos cardiopulmonares foram submetidos in situ a perfusão anterógrada com solução de baixo potássio e dextrana a 4°C via artéria pulmonar, extraídos em bloco e armazenados a 4°C por 6 h. Os blocos foram ventilados e perfundidos em um sistema ex vivo por 50 min, sendo obtidas medidas de mecânica ventilatória, hemodinâmica e trocas gasosas. Resultados: Houve redução da pressão arterial pulmonar média após a nebulização em todos os grupos (p < 0,001), sem diferença entre os grupos. Na perfusão ex vivo, a mecânica ventilatória não diferiu entre os grupos. Houve redução da capacidade relativa de oxigenação ao longo da perfusão nos grupos SN e SP (p = 0,04), e houve aumento significativo da pressão arterial pulmonar no grupo SN. Conclusões: O modelo experimental de administração de PGI2 na extração pulmonar é exequível e confiável. Na reperfusão, os resultados de hemodinâmica e de trocas gasosas demonstraram tendência a um melhor desempenho com o uso de PGI2 do que com solução salina.
Palavras-chave:
Prostaglandinas; Transplante de pulmão; Reperfusão; Modelos animais; Ratos.
IntroductionLung transplantation is a well-established therapeutic alternative for selected patients with end-stage lung disease. Lung function immediately after transplantation is affected by how well the donor lung was preserved, as is the subsequent performance of the organ.(1) Since the early days of lung preservation, prostanoids have been used in order to enhance preservation. Prostanoids have beneficial effects on graft function after reperfusion and are therefore added to intracellular solutions for preservation, such as the Euro-Collins and University of Wisconsin solutions. Because experimental animal studies have shown that prostaglandin E1 (PGE1) changes the cytokine profile from pro-inflammatory to anti-inflammatory,(2) it has been incorporated into lung preservation protocols as an adjuvant to extracellular solutions. However, the addition of prostanoids directly to the pulmonary circulation causes severe systemic hypotension, and prolonged hypotension can cause lung graft dysfunction, which is why prostanoids can only be used immediately before lung extraction.(3) Inhaled prostacyclin-prostaglandin I2 (PGI2)-has been used as a pulmonary vasodilator in the treatment of pulmonary hypertension and has the advantage of not causing systemic hypotension.(4) Therefore, prostacyclin can be administered to donors for prolonged periods, which increases the beneficial effects on the lung to be transplanted. There is still no consensus regarding the efficacy of inhaled prostanoids in preserving the lung for transplantation. This merits investigation, given that other inhaled agents, such as drugs and perfluorocarbons, have also been shown to be beneficial for the transplanted lung.(5,6)
The objective of the present study was to present a model of PGI2 administration (inhaled vs. parenteral) and to assess the functional performance of the lungs in an ex vivo lung perfusion system in rats.
MethodsAdult male Wistar rats (n = 40), weighing 300-350 g, were obtained from the animal facility of the University of São Paulo School of Medicine, in the city of São Paulo, Brazil. The animals were maintained under controlled temperature and humidity on a 12/12-h light/dark cycle. The animals had ad libitum access to water and Nuvilab CR-1 chow (Nuvital Nutrientes Ltda., Colombo, Brazil).
The study was approved by the local animal research ethics committee. The animals were anesthetized via inhalation of 5% isoflurane in a bell jar. They subsequently underwent tracheostomy and were mechanically ventilated with a flexiVent® ventilator (SCIREQ, Montreal, Canada). The animals were maintained under the following conditions: RR, 90 breaths/min; tidal volume (VT), 10 mL/kg; FiO2, 1.0; and 3% isoflurane. A median sterno-laparotomy was performed, and the animals received heparin (1,500 IU) through the inferior vena cava. The diaphragm was opened radially, and the inferior pulmonary ligament was sectioned. A 23-G scalp vein needle was inserted into the main pulmonary artery and was fixed into position. Mean pulmonary artery pressure (mPAP) was measured at baseline. The animals were ventilated for 2 min and were subsequently randomized into four groups (10 rats/group):
inhaled saline (IS) group-mechanical ventilation (2 min) + first nebulization with saline (1 min) + interval (30 s) + second nebulization with saline (1 min), mPAP being measured before and after nebulization
parenteral saline (PS) group-mechanical ventilation (2 min) + nebulization with saline (1 min) + interval (30 s) + second nebulization with saline (1 min) + administration of saline (0.1 mL) via the pulmonary artery, mPAP being measured before nebulization and after the administration of saline via the pulmonary artery
inhaled PGI2 (IPGI2) group-mechanical ventilation (2 min) + nebulization with PGI2 (Ilomedin; Schering, Traiskirchen, Austria) at 20 µg/kg (1 min) + interval (30 s) + second nebulization with PGI2 (1 min), mPAP being measured before and after nebulization
parenteral PGI2 (PPGI2) group-mechanical ventilation (2 min) + nebulization with saline (1 min) + interval (30 s) + second nebulization with saline (1 min) + administration of PGI2 (10 µg/kg) via the pulmonary artery, mPAP being measured before nebulization and after the administration of PGI2 via the pulmonary artery
Nebulization was performed with an ultrasonic nebulizer (Aeroneb®; Aerogen, Galway, Ireland) connected directly to the tracheostomy tube. In order to analyze total respiratory system resistance (Rrs) and compliance (Crs), we used the one-compartment model(7):
P = Rrs × VY + Crs × V + P0
where P is the airway opening pressure, Y is the flow, V is the volume, and P0 is the value of P when both Y and V are equal to zero, assuming a practical use in the absorption of errors in situations in which functional residual capacity is calculated on the basis of an integrated flow signal. The model was adjusted to a 1-s sine wave volume perturbation, with a VT of 10 mL/kg. Basal values were recorded before sternotomy, after sternotomy, and after nebulization.
After the second nebulization and the measurement of mPAP, the inferior vena cava was sectioned, the main pulmonary artery was cannulated, and a longitudinal left ventriculotomy was performed. The heart-lung blocks were submitted to perfusion with 20 mL of a low potassium and dextran solution (Perfadex®; Vitrolife, Kungsbacka, Sweden) at 4°C, the pressure being maintained at 10 cmH2O by raising the reservoir, and drainage of effluent through the left ventriculotomy, as previously described.(8,9) During perfusion with the preservation solution, the lungs were hyperventilated. After perfusion, the trachea was ligated to the partially inflated lungs, lung extraction was performed, and the heart-lung blocks were stored in saline for 6 h at 4-7°C.
We used an ex vivo perfusion circuit (IL2 - Isolated Perfused Rat or Guinea Pig Lung System; Harvard Apparatus, Holliston, MA, USA; Hugo Sachs Elektronik, Hugstetten, Germany) capable of measuring respiratory mechanics and hemodynamic parameters. The heart-lung blocks were perfused with a mixture of homologous blood and saline solution at a ratio of 1:1, in a total volume of approximately 80 mL, with a hematocrit of 15-20%. Pulmonary venous blood was deoxygenated with a membrane oxygenator (D150 MediSulfone® Hemofilter; MEDICA s.r.l. Medolla, Italy) containing a gas mixture (N2/CO2 at a ratio of 9:1) administered continuously (flow rate, 100 mL/min).
After a heart-lung block had been positioned in the ex vivo lung perfusion system, ventilation (RR, 60 breaths/min; inhalation/exhalation ratio, 60%; breath/min rate, 50% over the VT; and positive end-expiratory pressure, 1 cmH2O) was started with 50% of the VT, which was increased until it reached 10 mL/kg of body weight. The heart-lung block was initially perfused at a low flow rate (2 mL/min), which was progressively increased over the course of 5-10 min until it reached 5-7 mL/min. After the heart-lung block had become stable, we collected data related to hemodynamics, respiratory mechanics, pulmonary arterial blood gases, and pulmonary venous blood gases-every 10 min for 50 min. Blood samples were collected through the pulmonary arterial cannula and left atrial cannula for blood gas analysis (ABL 800; Radiometer, Copenhagen, Denmark). The relative oxygenation capacity of the lungs(10) was calculated by the following formula:
ROC = [(PvO2 − PaO2) × 100]/PaO2
where ROC is the relative oxygenation capacity, PaO2 is the deoxygenated blood collected through the pulmonary arterial cannula, and PvO2 is the oxygenated blood collected through the left atrial cannula. We corrected blood perfusate pH by adding sodium bicarbonate to the system (0.3 mEq L−1 dose−1) in order to maintain arterial pH within the 7.1-7.4 range.(11) After perfusion, the heart-lung block was removed, and the left lung was weighed and stored at 70°C for 72 h, after which it was weighed again in order to determine the wet/dry lung weight ratio.
We used descriptive statistics. Quantitative data with normal distribution are expressed as means and the respective standard errors. Quantitative data without normal distribution are expressed as medians. The assumptions of normal distribution in each group and homogeneity of variances among the groups were evaluated by the Shapiro-Wilk test and the Levene test, respectively. For quantitative dependent variables, we used repeated measures ANOVA. The Bonferroni test was used for multiple comparisons of the means. In the absence of normal distribution and homogeneity of variance, we used nonparametric tests (the Kruskal-Wallis test for the variable "type of solution" and the Mann-Whitney test for the variable "position"). The level of significance was set at p < 0.05. The analyses were performed with the Statistical Package for the Social Sciences, version 13.0 (SPSS Inc., Chicago, IL, USA).
ResultsThere were no significant differences among the four groups in terms of the mean weight of the animals (p > 0.05), which was 312 g in the IS group, 319 g in the PS group, 317 g in the IPGI2 group, and 317 g in the PPGI2 group.
For the sample as a whole, the mPAP was 11.4 ± 0.7 mmHg before nebulization and 8.1 ± 0.8 mmHg after (p < 0.001). The analysis of the difference between the pre- and post-nebulization mPAP revealed that nebulization significantly reduced mPAP in all of the groups (Table 1). Although the reduction in mPAP was more pronounced in the PPGI2 group than in the remaining groups, the difference was not statistically significant (p = 0.63). The evaluation of respiratory mechanics during mechanical ventilation showed that the four groups behaved similarly in terms of Rrs and Crs (Table 2).
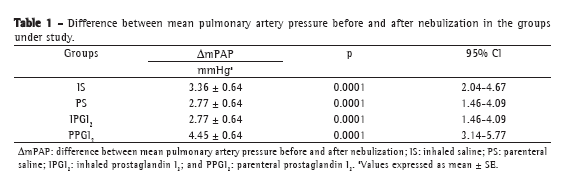
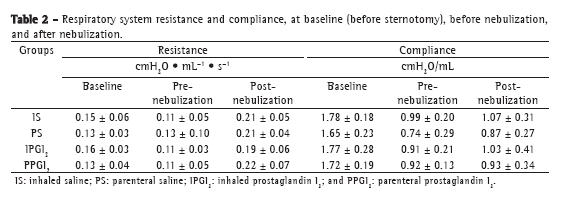
All of the lungs were perfused for 50 min in the ex vivo lung perfusion system, with the exception of two PS group lungs, which became unviable because of edema at 30 min and 40 min, respectively. There were no significant differences among the four groups regarding mean VT (p = 0.53), which was 1.45 mL in the IS group, 1.84 mL in the PS group, 1.93 mL in the IPGI2 group, and 1.66 mL in the PPGI2 group.
Among the PS, IPGI2, and PPGI2 groups, Rrs was similar. Although Rrs was higher in the IS group than in the remaining groups, the difference was not significant (p = 0.08).
Among the IS, PS, and PPGI2 groups, Crs was similar. In the latter half of the perfusion phase, Crs was slightly higher in the IPGI2 group, although the difference was not significant (p = 0.43).
There were no significant differences among the four groups under study regarding the relative oxygenation capacity of the lungs (p = 0.29). In the IS group, there was a significant decrease in the relative oxygenation capacity of the lungs measured at 50 min of perfusion when compared with that measured at the other perfusion time points (p = 0.01). The same occurred in the PS group, in which the relative oxygenation capacity of the lungs measured at 50 min of perfusion was lower than was that measured at 10 min (p = 0.04; Figure 1).
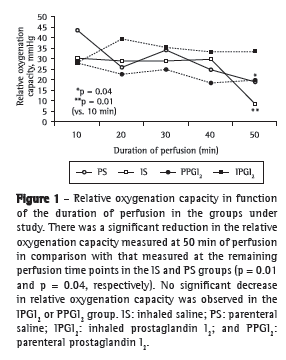
There were no significant differences among the four groups regarding mPAP (p = 0.11), which was 9.5 ± 1.1 mmHg in the IS group, 7.4 ± 1.3 mmHg in the PS group, 7.2 ± 1.1 mmHg in the IPGI2 group, and 5.3 ± 1.2 mmHg in the PPGI2 group. Although there was a progressive increase in mPAP over the course of the ex vivo lung perfusion in all of the groups, the increase was significant only in the IS group (p = 0.01; Figure 2).
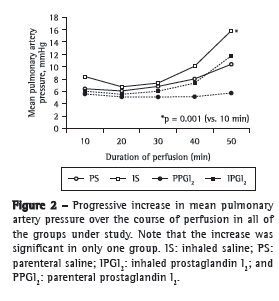
Over the course of the ex vivo perfusion, there was a gradual and significant increase in the weight of the heart-lung blocks (p = 0.001), the increase being more evident in the IS and PS groups at 40 min of perfusion. The differences among the groups were not significant (p = 0.59). Although the nominal values of the wet/dry lung weight ratio were higher in the IS and PS groups, there were no significant differences among the four groups regarding that aspect (p = 0.63; Figure 3).
DiscussionPrimary graft dysfunction (PGD) is a form of acute lung injury that results from a sequence of events occurring between the brain death of the donor and the reperfusion of the graft.(12) In addition to increasing morbidity and mortality, PGD increases the risk of acute rejection, impairing the long-term function of the transplanted lung. In deceased donors, increased IL-8 concentration in the BAL fluid and lung tissue correlates with the incidence of PGD.(13)
Hypothermia and the administration of an intracellular or extracellular solution play a fundamental role in lung preservation because they reduce the metabolic activity and degradation of cellular components and are implicated in the activation of inflammatory mediators.(14,15) Prostaglandins, such as PGE1 and PGI2, are potent vasodilators that are generally administered directly to the pulmonary circulation at the time of graft perfusion and are added to the hypothermic preservation solution. The beneficial effect of prostaglandins on lung transplantation is related to the preservation of the endothelial barrier of the pulmonary vasculature(16) and to vasodilation, which facilitates the distribution of the preservation solution through the lung parenchyma at extraction. Intracellular solutions, such as the Euro-Collins and University of Wisconsin solutions, have a high potassium content, which causes pulmonary vasoconstriction, vasodilators being therefore required during the administrations of such solutions. However, even after the introduction of low potassium extracellular solutions, prostaglandins continued to be used because their benefits go beyond vasodilation.(17) The fact that other vasodilators are not as efficient in preventing PGD supports the idea that the effects of prostaglandins are not limited to vasodilation.(2) Prostanoids are also able to ameliorate reperfusion injury through direct cytoprotection, effected by mediating the balance between pro- and anti-inflammatory cytokines. In rat models of lung transplantation, the administration of PGE1 during reperfusion increases IL-10 in lung tissue, whereas it decreases TNF-, IL-12, and IFN-.(18) However, prostanoids have not been found to be as effective in dog lungs harvested 3 h after cardiac arrest.(19) In the setting of extreme injury, the addition of PGE1 appears to have no beneficial effects.(19) Therefore, adding prostanoids to the pulmonary circulation might not be sufficient in that setting.
Clinically, the addition of high doses of prostanoids is limited to the moment of lung extraction, in order to minimize the notoriously deleterious effects of systemic arterial hypotension on the transplanted lung.(3) Inhalation has been proposed as an alternative method of selective pulmonary vasodilation without systemic effects. It has been demonstrated that inhaled PGI2 reduces mPAP and redistributes pulmonary flow without causing significant systemic hypotension.(20,21) The use of inhaled PGI2 in patients with ARDS has been reported to reduce pulmonary vascular resistance by 30%, improving gas exchange (by redistributing the perfusion to areas that were previously less perfused) without causing systemic hypotension.(22) Experimental studies have shown that the vasodilator properties of IPGI2 are as effective as are those of nitric oxide.(23) The use of inhaled PGI2 is currently recommended in cases of pulmonary hypertension, right ventricular failure, and ARDS, as well as in the treatment of congenital heart diseases associated with pulmonary hypertension.(22,24) A natural prostaglandin with a half-life of 6 min, PGI2 has antithrombotic properties and inhibits platelet aggregation, as well as having effects that are similar to those of nitric oxide. The use of inhaled PGI2 in patients with ARDS reduces pulmonary shunt fraction, improving oxygenation while reducing mPAP.(25)
On the basis of the abovementioned evidence, we can suggest that the administration of prostanoids through inhalation minimizes the systemic effects (therefore allowing prostanoids to be used for prolonged periods) and, at the same time, maximizes the beneficial effects on the donor lung. We used an ex vivo rat lung perfusion model in order to evaluate preservation methods. The ex vivo lung perfusion system has the advantage of serving as a screening method for new lung preservation strategies and has an excellent cost-benefit ratio.(8,9,11) Another group of authors used the same system in order to test preservation solutions and administer PGI2 parenterally.(10) The same authors recently demonstrated that the functional performance of transplanted lungs in pigs was better in the animals receiving PGI2 by inhalation than in those receiving PGI2 parenterally.(26) Those authors also demonstrated that PGI2 delivered via an ultrasonic nebulizer before lung extraction resulted in less edema, better gas exchange, and increased lung compliance.(27)
Our choice of a 6-h ischemia period was based on a previous study, in which we demonstrated that this duration of ischemia results in a larger number of viable lungs during perfusion.(11) Our decision to use a dose of 10 µg/kg when administering PGI2 parenterally was based on the dose used in another study (a mean of 10 µg every 3 min).(26) Because we found no reports of the dose of inhaled PGI2 to be used in models similar to ours, we empirically determined that the dose of inhaled PGI2 should be twice that of parenteral PGI2, in order to increase airway exposure to the drug and evaluate its systemic effects. In the study sample as a whole, there was a 29% reduction in mPAP after nebulization. Hypotension was more evident in the PPGI2 group, a finding that is in agreement with those reported for models involving larger animals.(28) Clinically, systemic hypotension has been reported to occur when inhaled PGI2 is used at a dose of 200-700 ng kg−1 min−1,(24) which corresponds to 20-80 µg of PGI2 administered over the course of 2 min to an adult weighing 60 kg.
Apparently, inhaled PGI2 does not interfere with respiratory mechanics (resistance and compliance) during lung extraction, as demonstrated by the similarities among our groups in terms of respiratory mechanics. Not interfering with respiratory mechanics is a desirable characteristic for lung preservation additives. The fact that PGI2 has this characteristic shows that its effects of are limited to the pulmonary vasculature and the cellular aspects of preservation.
In the present ex vivo lung perfusion study, functional performance was similar between the IPGI2 and PPGI2 groups, the former trending toward better lung compliance. Gas exchange was found to be worst in the IS group.
One characteristic of the ex vivo lung perfusion model used in the present study is an increase in mPAP over the course of perfusion, because the ex vivo lung perfusion system employs a nonpulsatile flow and an extracorporeal circuit. Beginning at 40 min of perfusion, there was an increase in mPAP and in the wet/dry lung weight ratio, which is suggestive of the presence of edema, in the IS and PS group lungs. Those increases, together with the fact that some of the PS group lungs became unviable before the end of the perfusion period, indicate that those lungs were not as well-preserved as were those in the IPGI2 and PPGI2 groups.
Although the nominal values of the wet/dry lung weight ratio were higher in the IS and PS groups, there were no significant differences among the four groups under study in terms of that ratio. This reflects a bias related to the ex vivo model, in which all of the lungs develop edema. This finding is possibly due to the combined effect of the increase in vascular permeability due to perfusion in an extracorporeal system and the 6-h duration of ischemia. Another group of authors used a similar system and found differences that were more pronounced among the groups; however, those authors limited the duration of hypothermic ischemia to 2-4 h.(10,29) An additional factor, which has recently come to our attention, is that Wistar rats can present with changes in vascular permeability due to an immune phenomenon related to allergy to dextran.(30) Because dextran is a compound that is found in the low potassium (Perfadex®) solution used in lung preservation, there will always be a possibility that that factor contributes to pulmonary edema. The use of a different breed, such as Sprague-Dawley, apparently reduces the likelihood of that type of allergic reaction.
In conclusion, the experimental model of PGI2 administration before lung extraction is feasible and reliable. During reperfusion, hemodynamics and gas exchange trended better with the use of PGI2 than with that of saline. The experimental model investigated in the present study can be used in future studies in order to evaluate other inhaled agents, such as perfluorocarbons.
References1. de Perrot M, Keshavjee S. Lung preservation. Semin Thorac Cardiovasc Surg. 2004;16(4):300-8.
2. Quadri SM, Segall L, de Perrot M, Han B, Edwards V, Jones N, et al. Caspase inhibition improves ischemia-reperfusion injury after lung transplantation. Am J Transplant. 2005;5(2):292-9.
3. Tremblay LN, Yamashiro T, DeCampos KN, Mestrinho BV, Slutsky AS, Todd TR, et al. Effect of hypotension preceding death on the function of lungs from donors with nonbeating hearts. J Heart Lung Transplant. 1996;15(3):260-8.
4. Machherndl S, Kneussl M, Baumgartner H, Schneider B, Petkov V, Schenk
P, et al. Long-term treatment of pulmonary hypertension with aerosolized iloprost. Eur Respir J. 2001;17(1):8-13.
5. Andrade CF, Martins LK, Tonietto TA, Koefender C, Anflor LC Jr, da Silva NB, et al. Partial liquid ventilation with perfluorodecalin following unilateral canine lung allotransplantation in non-heart-beating donors. J Heart Lung Transplant. 2004;23(2):242-51.
6. Ragaller M, Bleyl J, Tschö U, Winkler T, Regner M, Rasche S, et al. Effects of inhalation of perfluorocarbon aerosol on oxygenation and pulmonary function compared to PGI2 inhalation in a sheep model of oleic acid-induced lung injury. Intensive Care Med. 2001;27(5):889-97.
7. Cardoso PF, Spino M, Mayer E, Shi SQ, Pop RD, Puskas J, et al. Use of an isolated in situ canine lung perfusion model to evaluate dopamine clearance by the lung. J Pharmacol Exp Ther. 1991;259(3):1271-6.
8. Pêgo-Fernandes PM, Werebe Ede C, Cardoso PF, Pazetti R, Oliveira KA, Soares PR, et al. Experimental model of isolated lung perfusion in rats: technique and application in lung preservation studies. J Bras Pneumol. 2010;36(4):490-3.
9. Pêgo-Fernandes PM, Werebe E, Cardoso PF, Pazetti R, de Oliveira KA, Soares PR, et al. Experimental model of isolated lung perfusion in rats: first Brazilian experience using the IL-2 isolated perfused rat or guinea pig lung system. Transplant Proc. 2010;42(2):444-7.
10. Wittwer T, Wahlers T, Fehrenbach A, Elki S, Haverich A. Improvement of pulmonary preservation with Celsior and Perfadex: impact of storage time on early post-ischemic lung function. J Heart Lung Transplant. 1999;18(12):1198-201.
11. Soares PR, Braga KA, Nepomuceno NA, Pazetti R, Correia AT, Cardoso PF, et al. Comparison between Perfadex and locally manufactured low-potassium dextran solution for pulmonary preservation in an ex vivo isolated lung perfusion model. Transplant Proc. 2011;43(1):84-8.
12. de Perrot M, Liu M, Waddell TK, Keshavjee S. Ischemia-reperfusion-induced lung injury. Am J Respir Crit Care Med. 2003;167(4):490-511.
13. De Perrot M, Sekine Y, Fischer S, Waddell TK, McRae K, Liu M, et al. Interleukin-8 release during early reperfusion predicts graft function in human lung transplantation. Am J Respir Crit Care Med. 2002;165(2):211-5.
14. Keshavjee SH, Yamazaki F, Cardoso PF, McRitchie DI, Patterson GA, Cooper JD. A method for safe twelve-hour pulmonary preservation. J Thorac Cardiovasc Surg. 1989;98(4):529-34.
15. Fiser SM, Tribble CG, Long SM, Kaza AK, Kern JA, Jones DR, et al. Ischemia-reperfusion injury after lung transplantation increases risk of late bronchiolitis obliterans syndrome. Ann Thorac Surg. 2002;73(4):1041-7; discussion 1047-8.
16. Suttorp N, Weber U, Welsch T, Schudt C. Role of phosphodiesterases in the regulation of endothelial permeability in vitro. J Clin Invest. 1993;91(4):1421-8.
17. Mayer E, Puskas JD, Cardoso PF, Shi S, Slutsky AS, Patterson GA. Reliable eighteen-hour lung preservation at 4 degrees and 10 degrees C by pulmonary artery flush after high-dose prostaglandin E1 administration. J Thorac Cardiovasc Surg. 1992;103(6):1136-42.
18. de Perrot M, Fischer S, Liu M, Jin R, Bai XH, Waddell TK, et al. Prostaglandin E1 protects lung transplants from ischemia-reperfusion injury: a shift from pro- to anti-inflammatory cytokines. Transplantation. 2001;72(9):1505-12.
19. Kohmann J, Castro M, Silva U, Ruschel M, Madruga G, Felicetti J, et al. Efeito da prostaglandina E1 na viabilidade pulmonar de cadáver após transplante pulmonar unilateral em cães. J Pneumol. 1995;20(Suppl 3):145.
20. Wetzel RC. Aerosolized prostacyclin. In search of the ideal pulmonary vasodilator. Anesthesiology. 1995;82(6):1315-7.
21. Haraldsson A, Kieler-Jensen N, Nathorst-Westfelt U, Bergh CH, Ricksten SE. Comparison of inhaled nitric oxide and inhaled aerosolized prostacyclin in the evaluation of heart transplant candidates with elevated pulmonary vascular resistance. Chest. 1998;114(3):780-6.
22. Walmrath D, Schneider T, Pilch J, Grimminger F, Seeger W. Aerosolised prostacyclin in adult respiratory distress syndrome. Lancet. 1993;342(8877):961-2.
23. Walmrath D, Schermuly R, Pilch J, Grimminger F, Seeger W. Effects of inhaled versus intravenous vasodilators in experimental pulmonary hypertension. Eur Respir J. 1997;10(5):1084-92.
24. Siobal M. Aerosolized prostacyclins. Respir Care. 2004;49(6):640-52.
25. Walmrath D, Schneider T, Schermuly R, Olschewski H, Grimminger F, Seeger W. Direct comparison of inhaled nitric oxide and aerosolized prostacyclin in acute respiratory distress syndrome. Am J Respir Crit Care Med. 1996;153(3):991-6.
26. Wittwer T, Franke UF, Sandhaus T, Thiene M, Groetzner J, Strauch JT, et al. Preischemic iloprost application for improvement of graft preservation: which route is superior in experimental pig lung transplantation: inhaled or intravenous? Transplant Proc. 2007;39(5):1345-9.
27. Wittwer T, Franke UF, Ochs M, Sandhaus T, Schuette A, Richter S, et al. Inhalative pre-treatment of donor lungs using the aerosolized prostacyclin analog iloprost ameliorates reperfusion injury. J Heart Lung Transplant. 2005;24(10):1673-9.
28. Puskas JD, Cardoso PF, Mayer E, Shi S, Slutsky AS, Patterson GA. Equivalent eighteen-hour lung preservation with low-potassium dextran or Euro-Collins solution after prostaglandin E1 infusion. J Thorac Cardiovasc Surg. 1992;104(1):83-9.
29. Wittwer T, Wahlers T, Fehrenbach A, Cornelius JF, Elki S, Ochs M, et al. Combined use of prostacyclin and higher perfusate temperatures further enhances the superior lung preservation by Celsior solution in the isolated rat lung. J Heart Lung Transplant. 1999;18(7):684-92.
30. Koller ME, Reed RK. Increased negativity of interstitial fluid pressure in rat trachea in dextran anaphylaxis. J Appl Physiol. 1992;72(1):53-7.
* Study carried out in the Department of Cardiorespiratory Diseases, Thoracic Surgery Section; in Laboratório de Investigação Médica 61 (LIM-61, Laboratory for Medical Research 61), specializing in Experimental Thoracic Surgery; in Laboratório de Investigação Médica 20 (LIM-20, Laboratory for Medical Research 20), specializing in Experimental Therapy; at the Heart Institute of the University of São Paulo School of Medicine Hospital das Clínicas; and in the Biomedical Engineering Laboratory, University of São Paulo Polytechnic Institute, São Paulo, Brazil.
Correspondence to: Paulo Manuel Pêgo-Fernandes. InCor/Hospital das Clínicas da Faculdade de Medicina da Universidade de São Paulo, Rua Dr. Enéas de Carvalho Aguiar, 44, Bloco 2, 2º andar, Sala 9, Cerqueira Cesar, CEP 05403-000, São Paulo, SP, Brasil.
Tel. 55 11 3069-5248. E-mail: paulo.fernandes@incor.usp.br
Financial support: This study received financial support from the Fundação de Amparo à Pesquisa do Estado de São Paulo (FAPESP, São Paulo Research Foundation). Karina Fechini is the recipient of a Young Investigator Grant from FAPESP.
Submitted: 24 May 2011. Accepted, after review: 29 July 2011..
About the authorsPaulo Francisco Guerreiro Cardoso
Visiting Associate Professor. Department of Cardiorespiratory Diseases, Thoracic Surgery Section, University of São Paulo School of Medicine, São Paulo, Brazil.
Rogério Pazetti
Researcher I. Laboratório de Investigação Médica 61 (LIM-61, Laboratory for Medical Research 61), specializing in Experimental Thoracic Surgery, University of São Paulo School of Medicine, São Paulo, Brazil.
Henrique Takachi Moriya
Assistant Professor. Engineering Department, Sector of Telecommunications and Control Systems, University of São Paulo Polytechnic Institute, São Paulo, Brazil.
Paulo Manuel Pêgo-Fernandes
Tenured Professor. Department of Cardiorespiratory Diseases, University of São Paulo School of Medicine, São Paulo, Brazil.
Francine Maria de Almeida
Master's Student. Department of Clinical Medicine, University of São Paulo School of Medicine, São Paulo, Brazil.
Aristides Tadeu Correia
Research Technician. University of São Paulo School of Medicine, São Paulo, Brazil.
Karina Fechini
Biomedical Student, Universidade de Santo Amaro - UNISA, University of Santo Amaro - São Paulo, Brazil.
Fabio Biscegli Jatene
Full Professor. Thoracic Surgery Section, Department of Cardiorespiratory Diseases, University of São Paulo School of Medicine, São Paulo, Brazil.