ABSTRACT
Objective: To investigate the impact of impaired pulmonary function on patient-centered outcomes after hospital discharge due to severe COVID-19 in patients without preexisting respiratory disease. Methods: This is an ongoing prospective cohort study evaluating patients (> 18 years of age) 2-6 months after hospital discharge due to severe COVID-19. Respiratory symptoms, health-related quality of life, lung function, and the six-minute walk test were assessed. A restrictive ventilatory defect was defined as TLC below the lower limit of normal, as assessed by plethysmography. Chest CT scans performed during hospitalization were scored for the presence and extent of parenchymal abnormalities. Results: At a mean follow-up of 17.2 ± 5.9 weeks after the diagnosis of COVID-19, 120 patients were assessed. Of those, 23 (19.2%) reported preexisting chronic respiratory diseases and presented with worse lung function and exertional dyspnea at the follow-up visit in comparison with their counterparts. When we excluded the 23 patients with preexisting respiratory disease plus another 2 patients without lung volume measurements, a restrictive ventilatory defect was observed in 42/95 patients (44%). This subgroup of patients (52.4% of whom were male; mean age, 53.9 ± 11.3 years) showed reduced resting gas exchange efficiency (DLCO), increased daily-life dyspnea, increased exertional dyspnea and oxygen desaturation, and reduced health-related quality of life in comparison with those without reduced TLC (50.9% of whom were male; mean age, 58.4 ± 11.3 years). Intensive care need and higher chest CT scores were associated with a subsequent restrictive ventilatory defect. Conclusions: The presence of a restrictive ventilatory defect approximately 4 months after severe COVID-19 in patients without prior respiratory comorbidities implies worse clinical outcomes.
Keywords:
Post-acute COVID-19 syndrome; Respiratory function tests; Exercise test; Quality of life; Follow-up studies.
RESUMO
Objetivo: Investigar o impacto do comprometimento da função pulmonar nos desfechos centrados no paciente após a alta hospitalar em pacientes sem doenças respiratórias preexistentes que foram hospitalizados em virtude de COVID-19 grave. Métodos: Trata-se de um estudo prospectivo de coorte em andamento, no qual pacientes com COVID-19 grave (com idade > 18 anos) são avaliados 2-6 meses depois da alta hospitalar. Avaliamos os sintomas respiratórios, a qualidade de vida relacionada à saúde, a função pulmonar e a distância percorrida no teste de caminhada de seis minutos. A definição de distúrbio ventilatório restritivo foi CPT abaixo do limite inferior da normalidade na pletismografia. As imagens de TC de tórax realizadas durante a hospitalização foram avaliadas quanto à presença e extensão de alterações parenquimatosas. Resultados: Em média 17,2 ± 5,9 semanas depois do diagnóstico de COVID-19, foram avaliados 120 pacientes. Destes, 23 (19,2%) relataram doenças respiratórias crônicas preexistentes e apresentaram pior função pulmonar e maior dispneia aos esforços na consulta de acompanhamento quando comparados aos outros participantes. Quando excluímos os 23 pacientes com doenças respiratórias preexistentes e mais 2 pacientes (sem medidas de volumes pulmonares), observamos distúrbio ventilatório restritivo em 42/95 pacientes (44%). Esse subgrupo de pacientes (52,4% dos quais eram do sexo masculino, com média de idade de 53,9 ± 11,3 anos) apresentou menor eficiência das trocas gasosas (DLCO), maior dispneia na vida diária e dessaturação de oxigênio ao exercício e redução da qualidade de vida relacionada à saúde em comparação com aqueles sem redução da CPT (50,9% dos quais eram do sexo masculino, com média de idade de 58,4 ± 11,3 anos). A necessidade de terapia intensiva e pontuações mais altas no escore de alterações parenquimatosas na TC de tórax apresentaram relação com distúrbio ventilatório restritivo subsequente. Conclusões: A presença de distúrbio ventilatório restritivo aproximadamente 4 meses depois da COVID-19 grave em pacientes sem comorbidades respiratórias prévias implica piores desfechos clínicos.
Palavras-chave:
Síndrome pós-COVID-19 aguda; Testes de função respiratória; Teste de esforço; Qualidade de vida; Seguimentos.
INTRODUCTION Long-lasting respiratory symptoms and impaired pulmonary function have been increasingly recognized as post-COVID-19 sequelae.(1) Although the respiratory system is subject to major involvement during SARS, a substantial burden of health loss that spans several extrapulmonary systems has also been reported.(2) Perceived poor health status after COVID-19 was not related to respiratory sequelae (persistent chest imaging abnormalities) or disease severity in the acute phase in one study with a median follow-up of 75 days after diagnosis.(2) Conversely, impaired pulmonary function parameters have shown significant correlations with worse dyspnea, as assessed by the modified Medical Research Council (mMRC) dyspnea scale, and worse Medical Outcomes Study 36-Item Short-Form Health Survey (SF-36) physical functioning domain scores 45 days after hospital discharge.(3)
The impact of prior respiratory comorbidities on pulmonary function and its relationship with enduring respiratory complaints and health-related quality of life (HRQoL) was also less explored in previous studies. It is conceivable that patients with prior respiratory comorbidities should ideally be excluded for an unbiased analysis of the effects that pulmonary function sequelae of acute COVID-19 have on clinical outcomes.(4) Furthermore, the international standard recommendation to define lung function impairment (values below the lower limit of normal, i.e., the 5th percentile of a healthy population)(5) has not been consistently followed(1,6,7) or reported.(8)
We hypothesized that persistent pulmonary dysfunction after severe COVID-19 would further impair clinical outcomes in patients recovering from the disease. Therefore, our primary objective was to assess the impact of resting ventilatory impairment (i.e., values below the lower limit of normal) on general HRQoL, respiratory symptoms, and exercise performance after hospitalization for severe COVID-19 in patients without chronic respiratory disease. Secondary objectives were to compare these clinical outcomes between patients with and without chronic respiratory disease, and identify predictors during hospitalization on the subsequent presence of ventilatory impairment in the latter group of patients.
METHODS This is an ongoing single-center prospective cohort study including adult patients hospitalized for severe COVID-19 pneumonia between March 31, 2020 and November 23, 2021. The burden of comorbidities was assessed by calculating the Charlson Comorbidity Index.(9) All procedures were performed during a single study visit, which occurred 2-6 months after laboratory confirmation of SARS-CoV-2 infection. During the visit, the study participants underwent full pulmonary function testing and a six-minute walk test (6MWT). Subsequently, they completed questionnaires to evaluate HRQoL and respiratory symptoms, as well as symptoms of anxiety, depression, and posttraumatic stress disorder (PTSD). The main outcome measures were obtained from cross-sectional analysis of the aforementioned data. The clinical, laboratory, and chest imaging data obtained during hospitalization were collected from patient medical records. A semiquantitative scoring system(10) was used in order to assess lung involvement on the first chest CT scan performed during hospitalization. Each of the five lung lobes was visually scored on a scale of 0 to 5, with 0 indicating no involvement, 1 indicating an involvement of < 5%, 2 indicating an involvement of 5-25%, 3 indicating an involvement of 26-49%, 4 indicating an involvement of 50-75%, and 5 indicating an involvement > 75%. The total CT score was the sum of the individual lobar scores and ranged from 0 (no involvement) to 25 (maximum involvement). Two thoracic radiologists evaluated the chest CT images in a digital database system (IMPAX, version 8.1.2.SP&.1; Agfa HealthCare, Mortsel, Belgium), and final scores were determined by consensus.
The study was approved by the local research ethics committee (Protocol no. 2020-0169) and was performed in accordance with the Declaration of Helsinki. All participating patients gave written informed consent. The study protocol was registered at ClinicalTrials.gov (Identifier: NCT04410107).
Patients ≥ 18 years of age with laboratory-confirmed severe COVID-19 seen in the respiratory department just before discharge were invited to participate, constituting a convenience sample. Laboratory confirmation of SARS-CoV-2 infection was defined as a positive RT-PCR result from a nasal swab. Severe COVID-19 was defined as fever or suspected lower respiratory tract infection plus one of the following criteria: 1) respiratory rate > 30 breaths/min; 2) severe respiratory distress or SpO2 of ≤ 93% on room air; or 3) pulmonary infiltrates > 50% on chest imaging within 24-48 h of hospital admission.(11) Patients who were clinically unstable 2 months before enrollment, those who had active respiratory tract infection, and those who had any clinical condition precluding the performance of the study procedures were excluded.
Procedures Spirometry, body plethysmography, single-breath DLCO measurement, and impulse oscillometry were performed in accordance with the American Thoracic Society/European Respiratory Society standards, with the use of an automated system (MasterScreen™ PFT; CareFusion, Yorba Linda, CA, USA). The last hemoglobin value measured during hospitalization was used for DLCO correction. Spirometry, lung volumes, and DLCO parameters were expressed as absolute and percent predicted values, in accordance with the Global Lung Function Initiative reference values.(12-14) Impulse oscillometry measurements were also expressed as absolute and percent predicted values.(15) Obstructive ventilatory defect (a reduction in the FEV1/FVC ratio after bronchodilator administration), restrictive ventilatory defect (reduced TLC), and reduced DLCO were characterized by measurements below the lower limit of normal (i.e., below the −1.645 z-score).(5)
The 6MWT was performed indoors in a flat, 25-m corridor, in accordance with the latest European Respiratory Society/American Thoracic Society technical standards. All 6MWTs were performed at least 30 min after the pulmonary function tests. Continuous monitoring of SpO2 was performed with a pulse oximetry sensor (PureLight® 8000AA; Nonin Medical, Inc., Plymouth, MN, USA) connected to an oximeter. The six-minute walk distance was expressed as a percentage of the predicted value,(16) and values below the lower limit of normal defined reduced exercise capacity.
The mMRC dyspnea scale was used in order to grade dyspnea during activities of daily living, the levels of dyspnea being graded from 0 (absence of dyspnea during strenuous exercise) to 4 (too breathless to leave the house or breathless while dressing or undressing).(17) Cough and sputum production were assessed through an adapted translation of the American Thoracic Society respiratory symptoms questionnaire.(18)
General HRQoL was assessed with the SF-36. The SF-36 is a 36-item questionnaire divided into eight domains that measure social, physical, and mental health aspects. Each domain score ranges from 0 to 100, with higher scores reflecting better quality of life. (19) Reference values for the Brazilian population(20) were used for comparison with the values obtained in the present study.
Depression and anxiety symptoms were assessed by the Beck Depression Inventory (BDI) and the Beck Anxiety Inventory (BAI), respectively. The BDI and the BAI consist of 21 sets of statements about depression and anxiety symptoms in the last 7 days, rated on a 0-to-3 ordinal Likert scale.(21,22) A BDI > 14 and a BAI > 8 indicate some level of depression(23) and anxiety,(22) respectively.
PTSD was evaluated through the self-report PTSD Checklist, Civilian Version,(24) which comprises 17 items that assess three symptom groups during the previous month, on a scale of 1 to 5 (not at all to very much): reexperiences, avoidance behavior/emotional numbness, and increased arousal.(23) A score ≥ 3 (average) for any of the 17 items is considered clinically significant.(25)
Statistical analysis Data were analyzed with the Predictive Analytics Software package, version 18.0 (SPSS Inc., Chicago, IL, USA). The level of significance was set at p < 0.05. Normality was assessed by the Kolmogorov-Smirnov test. Continuous data were presented as mean ± SD or median (25th 75thpercentiles), depending on the data distribution. Categorical variables were reported as frequencies and proportions. Comparisons between groups were performed with the independent Student’s t-test, Pearson’s chi-square test, or Fisher’s exact test. Stepwise logistic regression analysis was used in order to identify hospitalization variables related to abnormal lung function in individuals without prior respiratory comorbidities.
RESULTS A total of 152 patients were evaluated for inclusion in the study. Of those, 120 (88.1%) were enrolled within a mean of 17.2 weeks of a positive RT-PCR test for SARS-CoV-2 infection (95% CI, 16.1-18.3; Figure 1). Twenty-three participants (19.2%) reported having chronic respiratory disease before COVID-19 (asthma, in 11; COPD, in 9; pulmonary tuberculosis, in 2; and bronchiectasis, in 1). Other comorbidities, daily-life dyspnea before COVID-19, duration of COVID-19 symptoms before admission, and hospitalization characteristics were similar between those with and without preexisting respiratory disease (data not shown). During the follow-up study visit, however, despite similar HRQoL and psychological symptoms, those with preexisting respiratory disease presented with worse lung function, exertional dyspnea, and oxygen saturation. Accordingly, the prevalences of obstructive ventilatory defect and abnormally reduced DLCO were higher (p < 0.001 and p = 0.10, respectively; Table 1), as were the prevalences of clinically relevant cough (4-6 times a day, ≥ 4 days a week; 31.8% vs. 11.0%; p = 0.01) and phlegm from the chest (> twice a day; 23.8% vs. 8.8%; p = 0.06).
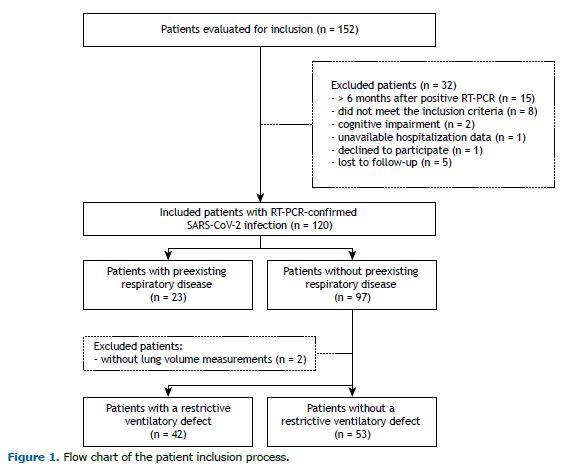
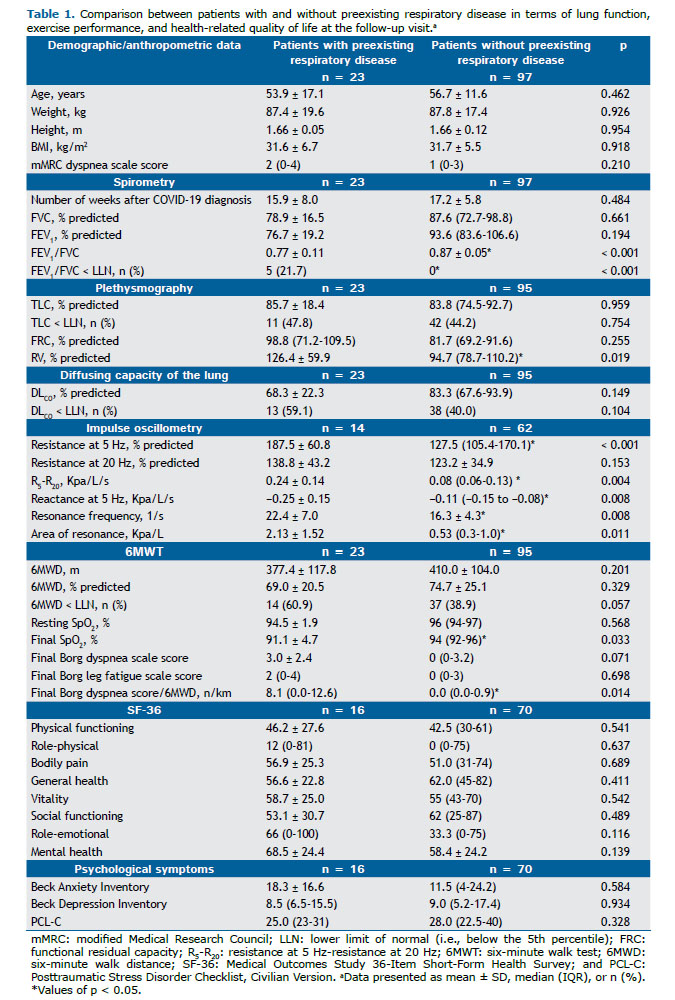
When we excluded patients with preexisting respiratory disease (n = 23) and those without lung volume measurements (n = 2), we observed a prevalence of 42/95 patients (44.2%) with restrictive disorder and a prevalence of 37/95 patients (38.9%) with abnormally reduced DLCO. Of the 42 patients with restrictive ventilatory impairment, 14 (33.3%) did not show reduced DLCO. None was found to have an obstructive ventilatory defect (with the use of the lower limit of normal or a fixed ratio below 0.7). Patients with a restrictive ventilatory defect had a shorter duration of symptoms before hospitalization and higher proportions of ICU admission and invasive mechanical ventilation, as well as longer length of hospital stay, longer duration of invasive mechanical ventilation, and higher chest CT scores. During the follow-up study visit, these patients presented with worse daily-life dyspnea and DLCO, as well as higher prevalences of reduced exercise capacity, exertional dyspnea, and oxygen desaturation (Table 2). General HRQoL was worse in almost all domains, the exceptions being bodily pain, role-emotional, and mental health (Figure 2). Although the prevalence of significant dyspnea (i.e., an mMRC dyspnea scale score ≥ 2) was higher in those with a restrictive ventilatory defect, there were no differences between those with and those without a restrictive ventilatory defect in terms of clinically relevant cough and sputum production (Figure 3). Regarding psychological symptoms, only anxiety-related complaints were higher in the former group of patients (Table 2).
Stepwise multivariate logistic regression analysis including age, sex, and the Charlson Comorbidity Index revealed that intensive care need and the magnitude of pulmonary involvement (as assessed by chest CT scores) predicted the presence of a restrictive ventilatory defect at the follow-up visit (Table 3).
DISCUSSION Persistent respiratory symptoms and impaired pulmonary function have been increasingly recognized as post-acute COVID-19 sequelae.(1) The extent to which preexisting respiratory conditions and impaired lung function after COVID-19 affect clinical outcomes is less clearly established. The present study showed that prior respiratory disease implied lower lung function and worse respiratory symptoms. By excluding these patients, we demonstrated that a restrictive ventilatory defect was a common finding (in 44%), usually associated with impaired gas exchange (¯DLCO) a few months (≈ 4 months) after hospitalization for severe COVID-19. A restrictive ventilatory defect was associated with negative effects on patient-centered outcomes, including exertional and daily-life dyspnea (an mMRC dyspnea scale score ≥ 2), as well as exercise capacity and general HRQoL.
Fatigue, dyspnea, and cough are among the most common complaints in studies examining symptoms after hospitalization or disease onset.(26) Interestingly, the aforementioned symptoms are also highly prevalent among patients not hospitalized during the acute phase. One recent study showed that persistent dyspnea after COVID-19 (13% of the sample had been hospitalized) was not associated with overt cardiopulmonary impairment or exercise intolerance.(27) Conversely, persistent dyspnea, fatigue, and exercise intolerance have been associated with peripheral oxygen delivery/utilization mismatch, respiratory limitation, or dysfunctional breathing in samples with hospital admission rates ranging from 10% to 96% during the acute phase of COVID-19.(28) In a study including only noncritically ill patients (27% of whom had no hypoxemia and were treated on an outpatient basis), those with persistent dyspnea were found to have lower FVC, lower DLCO, lower six-minute walk distance, and increased exertional desaturation,(29) findings that are consistent with ours. However, the authors of the aforementioned study(29) did not quantify the severity of persistent dyspnea or measure TLC in order to diagnose a restrictive ventilatory defect. A reduced FVC and/or FEV1 with normal FEV1/FVC are suggestive of a restrictive pattern, and lung volume measurements are usually needed in order to confirm that. Restriction is a potential dysfunction following severe lung injury (and related medical care) caused by parenchymal abnormalities (interstitial lung disease) or extraparenchymal abnormalities (respiratory muscle wasting resulting in atrophy and weakness). (5) A low DLCO in the presence of reduced TLC (and alveolar volume) may result from any one of the aforementioned mechanisms. Meanwhile, reduced DLCO in patients without restriction (or obstruction) indicates impaired gas exchange efficiency, which, in the present context, indicates either interstitial lung disease (alveolar destruction, alveolar thickening, or ventilation/perfusion mismatch) without mechanical abnormalities, or it indicates pulmonary vascular disease.(30) Finally, the presence of reduced TLC with preserved DLCO indicates extraparenchymal restriction. (5) The complexity of interpreting DLCO, lung volume (or rather, alveolar volume), and the relationship between the two, however, makes it difficult to determine the underlying abnormality (or abnormalities) on the basis of the available data.(31) Respiratory muscle evaluation and more specific measures of the alveolar-capillary membrane (e.g., combined DLCO and diffusing capacity of the lung for nitric oxide measurements or advanced chest imaging techniques) might be useful to assess the contribution of interstitial lung abnormalities, pulmonary vascular injury, and/or extraparenchymal abnormalities to reduced DLCO in individual cases.(31)
The roles that acute disease severity and the extent of pneumonia on chest CT scans play in the development of impaired lung function and respiratory symptoms are also contradictory. A significant relationship between disease severity and abnormal lung function has been reported in some studies,(6,29) but not in others.(32) In agreement with the findings of a study assessing risk factors for post-COVID-19 pulmonary fibrosis,(33) our findings show that the need for intensive care and a greater extent of acute pulmonary inflammation (i.e., a higher chest CT score) independently predicted restrictive lung disease at the follow-up visit.
Psychological stress is highly prevalent after COVID-19.(34) It is associated with breathlessness and poorer functional status in the general population.(35) In the present study, self-report questionnaires were used in order to assess the modulation of PTSD, depression, and anxiety symptoms in the relationship between impaired respiratory function and worse clinical outcomes. Mean BDI scores indicated mild to no depression. Although PTSD symptoms were clinically significant, there were no differences between patients with and without a restrictive ventilatory defect. Anxiety symptoms were significantly higher in the former group of patients, raising the question of the contribution of anxiety symptoms to worsening clinical outcomes. Regardless of the direction of this relationship (cause or consequence), it seems valuable to assess and manage anxiety symptoms, and further research is needed in order to explore the impact of anxiety management on post-COVID-19 symptom relief.
The prevalences of asthma and COPD before COVID-19 have been reported to range from 5% to 15% and from 1% to 9%, respectively.(26) Of the patients in our sample, 19% reported baseline respiratory comorbidities and were excluded from later analyses. Significant dyspnea (an mMRC dyspnea scale score ≥ 2) was more common in patients with restrictive lung disease than in those without it (57% vs. 24%). The overall proportion of patients presenting with clinically significant cough and sputum production was not negligible for patients without preexisting respiratory conditions (i.e., 8-12%). However, this rate was not worse in the presence of a restrictive ventilatory defect. Cough and expectoration are typical symptoms of airway inflammation secondary to tracheobronchitis or pneumonia. Viral respiratory tract infections can be associated with acute bronchiolitis in adults, and constrictive bronchiolitis can be seen as a late sequela of viral lower respiratory tract infections.(36) The prevalence of an obstructive ventilatory pattern after COVID-19 was reported to be 7% in a previous systematic review. (1) However, differences across studies regarding the criteria to define obstruction and the presence of prior chronic respiratory disease justify caution in considering persistent expiratory airflow obstruction to be a late sequela of COVID-19. For example, of the studies included in the aforementioned systematic review,(1) only one(32) used the recommended lower limit of normal to define obstruction, and none of the patients in that study had an obstructive ventilatory pattern. In another study using impulse oscillometry measurements, mean values of the respiratory system resistance at an oscillation frequency of 5 Hz and of 20 Hz were reported to be normal 30 days after hospital discharge (127 ± 29% of predicted and 133 ± 31% of predicted, respectively).(37) As expected, the prevalence of obstructive lung disease was higher in our patients with preexisting respiratory disease (i.e., 21.7%), and they had worse impulse oscillometry measurements than did those without preexisting respiratory disease. Although mean values of respiratory system resistance at an oscillation frequency of 5 Hz and of 20 Hz were within the normal range in our patients without preexisting respiratory disease, the mean resonance frequency and area of resonance were altered. These findings suggest the involvement of peripheral lung parenchyma without airway disease.(38)
From a clinical perspective, we demonstrated that persistent lung function impairment implies worse dyspnea and physical functioning after COVID-19, mainly represented by a restrictive ventilatory defect associated with abnormal gas exchange. Therefore, pulmonary function testing might be useful to uncover factors contributing to such complaints; to monitor changes over time; and to guide future studies aimed at evaluating potential interventions for their relief.
One of the limitations of the present study is the lack of assessment of other potential mechanisms for respiratory symptoms and impaired HRQoL after COVID-19. Cardiac dysfunction, musculoskeletal dysfunction, immune response to SARS-CoV-2,(34) and impaired oxidative metabolism(39) have been suggested to explain dyspnea, fatigue, and exercise intolerance beyond alterations in pulmonary function. Another limitation is the relatively short-term follow-up. Although data from long-term follow-up of previous coronavirus outbreaks(40) and 12-month follow-up of the current COVID-19 pandemic(6) suggest that some patients will have long-term respiratory complications, improvement in pulmonary function is commonly observed over time. (6) Nevertheless, this is an ongoing prospective cohort study that will provide long-term (> 12-month) follow-up of pulmonary function, exercise capacity, HRQoL, and their interplay. Finally, we do not know whether our findings apply to cases of milder disease, because only patients hospitalized for severe COVID-19 pneumonia were included in the present study.
In this prospective cohort of patients followed for approximately 4 months after a confirmed diagnosis of severe COVID-19, those with preexisting chronic respiratory comorbidities showed worse lung function and respiratory symptoms. When those patients were excluded, poor resting gas exchange (reduced DLCO), increased dyspnea, reduced HRQoL, and reduced exercise performance were observed in those with a restrictive ventilatory defect. Intensive care need and a greater extent of pulmonary involvement during the acute phase were associated with the presence of a restrictive ventilatory defect at the follow-up visit.
AUTHOR CONTRIBUTIONS DCB, MBG, PTRD, and SPR: study conception/design and literature review. IGB, RMCS, GMH, GSV, and ARG: data collection. LF, VBB, and TSG: analysis and interpretation of chest CT scans. DCB, IGB, and RMCS: statistical analysis and interpretation. DCB, RMCS, GMH, and ARG: drafting of the first version of the manuscript. All authors critically reviewed and approved the final version of the manuscript.
CONFLICTS OF INTEREST None declared.
REFERENCES 1. Torres-Castro R, Vasconcello-Castillo L, Alsina-Restoy X, Solis-Navarro L, Burgos F, Puppo H, et al. Respiratory function in patients post-infection by COVID-19: a systematic review and meta-analysis. Pulmonology. 2021;27(4):328-337. https://doi.org/10.1016/j.pulmoe.2020.10.013
2. Townsend L, Dowds J, O’Brien K, Sheill G, Dyer AH, O’Kelly B, et al. Persistent Poor Health after COVID-19 Is Not Associated with Respiratory Complications or Initial Disease Severity. Ann Am Thorac Soc. 2021;18(6):997-1003. https://doi.org/10.1513/AnnalsATS.202009-1175OC
3. van der Sar-van der Brugge S, Talman S, Boonman-de Winter L, de Mol M, Hoefman E, van Etten RW, et al. Pulmonary function and health-related quality of life after COVID-19 pneumonia. Respir Med. 2021;176:106272. https://doi.org/10.1016/j.rmed.2020.106272
4. Huang L, Li X, Gu X, Zhang H, Ren L, Guo L, et al. Health outcomes in people 2 years after surviving hospitalisation with COVID-19: a longitudinal cohort study. Lancet Respir Med. 2022;10(9):863-876. https://doi.org/10.1016/S2213-2600(22)00126-6
5. Stanojevic S, Kaminsky DA, Miller MR, Thompson B, Aliverti A, Barjaktarevic I, et al. ERS/ATS technical standard on interpretive strategies for routine lung function tests. Eur Respir J. 2022;60(1):2101499. https://doi.org/10.1183/13993003.01499-2021
6. Wu X, Liu X, Zhou Y, Yu H, Li R, Zhan Q, et al. 3-month, 6-month, 9-month, and 12-month respiratory outcomes in patients following COVID-19-related hospitalisation: a prospective study. Lancet Respir Med. 2021;9(7):747-754. https://doi.org/10.1016/S2213-2600(21)00174-0
7. Faverio P, Luppi F, Rebora P, Busnelli S, Stainer A, Catalano M, et al. Six-Month Pulmonary Impairment after Severe COVID-19: A Prospective, Multicentre Follow-Up Study. Respiration. 2021;100(11):1078-1087. https://doi.org/10.1159/000518141
8. Abdallah SJ, Voduc N, Corrales-Medina VF, McGuinty M, Pratt A, Chopra A, et al. Symptoms, Pulmonary Function, and Functional Capacity Four Months after COVID-19. Ann Am Thorac Soc. 2021;18(11):1912-1917. https://doi.org/10.1513/AnnalsATS.202012-1489RL
9. Charlson ME, Pompei P, Ales KL, MacKenzie CR. A new method of classifying prognostic comorbidity in longitudinal studies: development and validation. J Chronic Dis. 1987;40(5):373-383. https://doi.org/10.1016/0021-9681(87)90171-8
10. Pan F, Ye T, Sun P, Gui S, Liang B, Li L, et al. Time Course of Lung Changes at Chest CT during Recovery from Coronavirus Disease 2019 (COVID-19). Radiology. 2020;295(3):715-721. https://doi.org/10.1148/radiol.2020200370
11. World Health Organization [homepage on the Internet]. Geneva: WHO; c2020 [updated 2020 Mar 13; cited 2022 Dec 1]. Clinical management of severe acute respiratory infection (SARI) when COVID-19 disease is suspected. Interim guidance. Available from: https://apps.who.int/iris/handle/10665/331446 https://doi.org/10.15557/PiMR.2020.0003
12. Quanjer PH, Stanojevic S, Cole TJ, Baur X, Hall GL, Culver BH, et al. Multi-ethnic reference values for spirometry for the 3-95-yr age range: the global lung function 2012 equations. Eur Respir J. 2012;40(6):1324-1343. https://doi.org/10.1183/09031936.00080312
13. Hall GL, Filipow N, Ruppel G, Okitika T, Thompson B, Kirkby J, et al. Official ERS technical standard: Global Lung Function Initiative reference values for static lung volumes in individuals of European ancestry. Eur Respir J. 2021;57(3):2000289. https://doi.org/10.1183/13993003.00289-2020
14. Stanojevic S, Graham BL, Cooper BG, Thompson BR, Carter KW, Francis RW, et al. Official ERS technical standards: Global Lung Function Initiative reference values for the carbon monoxide transfer factor for Caucasians [published correction appears in Eur Respir J. 2020 Oct 15;56(4):]. Eur Respir J. 2017;50(3):1700010. https://doi.org/10.1183/13993003.00010-2017
15. Vogel J, Smidt U. Impulse Oscillometry. Analysis of lung mechanics in general practice and clinic, epidemiological and experimental research. Frankfurt: PMI-Verlagsgruppe; 1994.
16. Britto RR, Probst VS, de Andrade AF, Samora GA, Hernandes NA, Marinho PE, et al. Reference equations for the six-minute walk distance based on a Brazilian multicenter study. Braz J Phys Ther. 2013;17(6):556-563. https://doi.org/10.1590/S1413-35552012005000122
17. Bestall JC, Paul EA, Garrod R, Garnham R, Jones PW, Wedzicha JA. Usefulness of the Medical Research Council (MRC) dyspnoea scale as a measure of disability in patients with chronic obstructive pulmonary disease. Thorax. 1999;54(7):581-586. https://doi.org/10.1136/thx.54.7.581
18. Comstock GW, Tockman MS, Helsing KJ, Hennesy KM. Standardized respiratory questionnaires: comparison of the old with the new. Am Rev Respir Dis. 1979;119(1):45-53.
19. Ciconelli RM, Ferraz MB, Santos W, Meinão I, Quaresma MR. Tradução para a língua portuguesa e validação do questionário genérico de avaliação de qualidade de vida SF-36 (Brasil SF-36) [Article in Portuguese]. Rev Bras Reumatol. 1999;39(3):143-150.
20. Laguardia J, Campos MR, Travassos C, Najar AL, Anjos LA, Vasconcellos MM. Brazilian normative data for the Short Form 36 questionnaire, version 2. Rev Bras Epidemiol. 2013;16(4):889-897. https://doi.org/10.1590/S1415-790X2013000400009
21. Gomes-Oliveira MH, Gorenstein C, Lotufo Neto F, Andrade LH, Wang YP. Validation of the Brazilian Portuguese version of the Beck Depression Inventory-II in a community sample. Braz J Psychiatry. 2012;34(4):389-394. https://doi.org/10.1016/j.rbp.2012.03.005
22. Rector N, Arnold P. Assessment of Patients with Anxiety Disorders. In: Goldbloom D, editor. Psychiatric Clinical Skills. Maryland Heights: Mosby; 2006. p. 71-89. https://doi.org/10.1016/B978-0-323-03123-3.50012-0
23. American Psychiatric Association. Diagnostic and statistical manual of mental disorders. 4th edition (DSM-IV). Washington DC: American Psychiatric Press; 1994.
24. Grubaugh AL, Elhai JD, Cusack KJ, Wells C, Frueh BC. Screening for PTSD in public-sector mental health settings: the diagnostic utility of the PTSD checklist. Depress Anxiety. 2007;24(2):124-129. https://doi.org/10.1002/da.20226
25. Berger W, Mendlowicz MV, Souza WF, Figueira I. Semantic equivalence of the Portuguese version of the Post-Traumatic Stress Disorder Checklist - Civilian Version (PCL-C) for the screening of post-traumatic stress disorder. Rev psiquiatr Rio Gd Sul. 2004;26(2):167-175. https://doi.org/10.1590/S0101-81082004000200006
26. Fernández-de-Las-Peñas C, Palacios-Ceña D, Gómez-Mayordomo V, Florencio LL, Cuadrado ML, Plaza-Manzano G, et al. Prevalence of post-COVID-19 symptoms in hospitalized and non-hospitalized COVID-19 survivors: A systematic review and meta-analysis. Eur J Intern Med. 2021;92:55-70. https://doi.org/10.1016/j.ejim.2021.06.009
27. Beaudry RI, Brotto AR, Varughese RA, de Waal S, Fuhr DP, Damant RW, et al. Persistent dyspnea after COVID-19 is not related to cardiopulmonary impairment; a cross-sectional study of persistently dyspneic COVID-19, non-dyspneic COVID-19 and controls. Front Physiol. 2022;13:917886. https://doi.org/10.3389/fphys.2022.917886
28. Frésard I, Genecand L, Altarelli M, Gex G, Vremaroiu P, Vremaroiu-Coman A, et al. Dysfunctional breathing diagnosed by cardiopulmonary exercise testing in ‘long COVID’ patients with persistent dyspnoea. BMJ Open Respir Res. 2022;9(1):e001126. https://doi.org/10.1136/bmjresp-2021-001126
29. Cortés-Telles A, López-Romero S, Figueroa-Hurtado E, Pou-Aguilar YN, Wong AW, Milne KM, et al. Pulmonary function and functional capacity in COVID-19 survivors with persistent dyspnoea. Respir Physiol Neurobiol. 2021;288:103644. https://doi.org/10.1016/j.resp.2021.103644
30. Neder JA, Berton DC, O’Donnell DE. Integrating measurements of pulmonary gas exchange to answer clinically relevant questions. J Bras Pneumol. 2020;46(1):e20200019. https://doi.org/10.1590/1806-3713/e20200019
31. Chapman DG, Badal T, King GG, Thamrin C. Caution in interpretation of abnormal carbon monoxide diffusion capacity in COVID-19 patients. Eur Respir J. 2021;57(1):2003263. https://doi.org/10.1183/13993003.03263-2020
32. Frija-Masson J, Debray MP, Gilbert M, Lescure FX, Travert F, Borie R, et al. Functional characteristics of patients with SARS-CoV-2 pneumonia at 30 days post-infection. Eur Respir J. 2020;56(2):2001754. https://doi.org/10.1183/13993003.01754-2020
33. Aul DR, Gates DJ, Draper DA, Dunleavy DA, Ruickbie DS, Meredith DH, et al. Complications after discharge with COVID-19 infection and risk factors associated with development of post-COVID pulmonary fibrosis. Respir Med. 2021;188:106602. https://doi.org/10.1016/j.rmed.2021.106602
34. Ramakrishnan RK, Kashour T, Hamid Q, Halwani R, Tleyjeh IM. Unraveling the Mystery Surrounding Post-Acute Sequelae of COVID-19. Front Immunol. 2021;12:686029. https://doi.org/10.3389/fimmu.2021.686029
35. Currow DC, Chang S, Reddel HK, Kochovska S, Ferreira D, Kinchin I, et al. Breathlessness, Anxiety, Depression, and Function-The BAD-F Study: A Cross-Sectional and Population Prevalence Study in Adults. J Pain Symptom Manage. 2020;59(2):197-205.e2. https://doi.org/10.1016/j.jpainsymman.2019.09.021
36. Ryu JH, Azadeh N, Samhouri B, Yi E. Recent advances in the understanding of bronchiolitis in adults. F1000Res. 2020;9:F1000 Faculty Rev-568. https://doi.org/10.12688/f1000research.21778.1
37. Huang Y, Tan C, Wu J, Chen M, Wang Z, Luo L, et al. Impact of coronavirus disease 2019 on pulmonary function in early convalescence phase. Respir Res. 2020;21(1):163. https://doi.org/10.1186/s12931-020-01429-6
38. Oostveen E, MacLeod D, Lorino H, Farré R, Hantos Z, Desager K, et al. The forced oscillation technique in clinical practice: methodology, recommendations and future developments. Eur Respir J. 2003;22(6):1026-1041. https://doi.org/10.1183/09031936.03.00089403
39. de Boer E, Petrache I, Goldstein NM, Olin JT, Keith RC, Modena B, et al. Decreased Fatty Acid Oxidation and Altered Lactate Production during Exercise in Patients with Post-acute COVID-19 Syndrome. Am J Respir Crit Care Med. 2022;205(1):126-129. https://doi.org/10.1164/rccm.202108-1903LE
40. Ngai JC, Ko FW, Ng SS, To KW, Tong M, Hui DS. The long-term impact of severe acute respiratory syndrome on pulmonary function, exercise capacity and health status. Respirology. 2010;15(3):543-550. https://doi.org/10.1111/j.1440-1843.2010.01720.x