A COVID-19 longa ou síndrome pós-COVID-19 é definida pela persistência de sintomas após quatro semanas do início do quadro viral, na ausência de replicação viral após 3 semanas.(1) Diversos estudos apontaram que em torno de 60-70% dos pacientes relatam persistência de sintomas por semanas a meses após o quadro agudo, sendo os principais: fadiga/fraqueza muscular, dispneia, depressão/ansiedade, alterações do sono e cognitivas. Não sabemos ainda porque uma parcela dos pacientes persiste com sintomas crônicos e porque a cronificação dos sintomas não necessariamente se correlaciona com a gravidade da infecção aguda.(2,3) As principais hipóteses estão relacionadas à própria toxicidade viral, alterações no sistema imune e da resposta inflamatória sistêmica, lesão endotelial e injúria microvascular e/ou microtrombos, proliferação de fibroblastos decorrente do dano alveolar difuso, além de lesões relacionadas à ventilação mecânica, uso de medicações (corticoides, bloqueadores neuromusculares, etc.), internação prolongada com imobilidade, e a síndrome de stress pós-traumático.
Frente a este cenário de cronificação dos sintomas, seria esperado, do ponto de vista fisiopatológico, impacto na tolerância ao exercício com redução da capacidade aeróbica. Mas o que nos mostra a literatura? Nos poucos estudos com teste de exercício cardiopulmonar (TECP) em COVID19 publicados até o momento (Tabela 1), foi sugerido que a intolerância ao exercício seria decorrente do descondicionamento (Tabela 1), foi sugerido que a intolerância ao exercício seria decorrente do descondicionamento físico.(4-6) Mas o que é descondicionamento? No dicionário médico é definido por “perda de aptidão física devido à incapacidade de manter um nível ideal de atividade física ou treinamento. A inatividade por qualquer motivo pode levar ao descondicionamento”. Já no TECP, e do ponto de vista fisiopatológico, é considerado como a redução do V’O2 pico, com ou sem um padrão leve de taquicardia, na ausência de doenças cardiocirculatórias centrais e periféricas conhecidas. A presença de limiar de lactato precoce, por exemplo, só está presente em indivíduos sem doenças cardiocirculatórias centrais que são extremamente sedentários e com alto comprometimento muscular pela inatividade, como no caso de pacientes com doenças crônicas debilitantes.
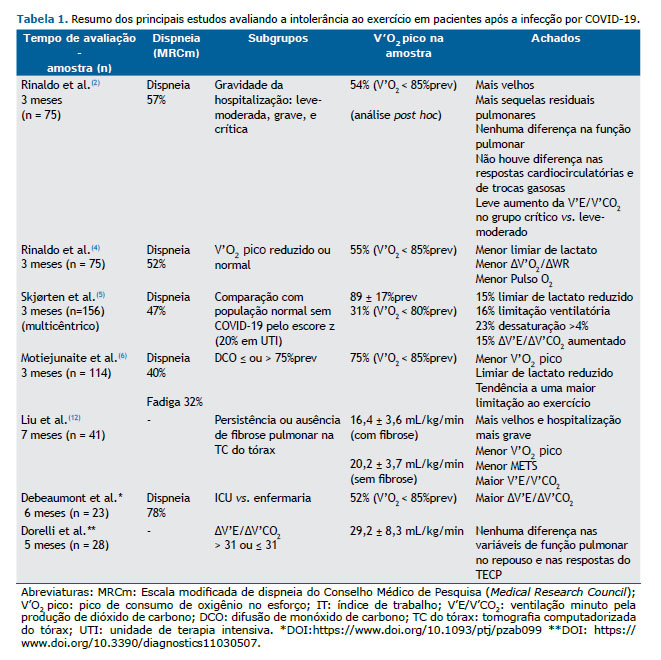
Quando falamos em avaliação de mecanismos de intolerância ao esforço com o uso de TECP é importante definirmos se a limitação ao esforço é de origem cardiocirculatória central ou periférica, e se há uma limitação ventilatória ou de trocas gasosas isolada ou associada. A limitação ao esforço de origem cardiocirculatória central, por exemplo, pode ocorrer mesmo na presença de exames cardiológicos de repouso normais, e pode estar relacionada à baixa oferta de O2. Levando-se em consideração a hipotética presença de miocardite e lesão endotelial/microvascular pulmonar na fase aguda da infecção por COVID-19, a limitação ao esforço de origem cardiovascular central na síndrome pós-COVID-19 poderia ser decorrente de lesão miocárdica inflamatória crônica - a prevalência de miocardite clínica e subclínica em atletas universitários foi 2,3% por ressonância cardíaca e pode ser um dos motivos de redução de desempenho nesta população(7) - ou de lesão microvascular pulmonar. A doença vascular pulmonar, detectada exclusivamente sob estresse físico, é também conhecida como ‘hipertensão pulmonar de esforço’. Além do comprometimento da bomba cardíaca, a oferta de O2 poderia ainda estar comprometida por redução do transporte de O2 em decorrência de anemia, principalmente após a alta hospitalar.(8)
Do ponto de vista periférico, a intolerância ao esforço pode ser decorrente do comprometimento da utilização periférica de O2 ou da redução da extração periférica de O2 por injúria mitocondrial, com consequente impacto negativo na produção de energia durante a respiração celular para formação de ATP. Neste contexto, Baratto et al.(8) demonstraram que os pacientes pós-COVID-19 na alta hospitalar apresentavam maior débito cardíaco (DC) em repouso, menor conteúdo arterial de O2 (transporte convectivo de O2 reduzido) e uma menor diferença arteriovenosa de O2 em relação a controles saudáveis, mas com taxa de extração semelhante. Durante o exercício, apesar do maior DC, os pacientes pós-COVID-19 apresentavam menor extração muscular de O2 na ausência de aumento da pressão de artéria pulmonar e resistência vascular pulmonar, justificando o menor V’O2 pico.(8) Ao avaliar pacientes com sintomas persistentes após infecção por COVID-19, Singh et al.(3) elegantemente demonstraram, por meio de TECP invasivo, que a oferta de O2 encontrava-se normal e associada a uma redução na taxa de extração periférica de O2 e elevação da saturação venosa mista de O2 em relação aos controles, resultando em um V’O2 pico reduzido e indicando uma menor oferta difusiva de O2 para as mitocôndrias.(3) Neste estudo, nenhum paciente apresentou limitação cardiocirculatória central. Corroborando o comprometimento muscular periférico por disfunção da respiração celular mitocondrial, e não por descondicionamento muscular periférico, um relato de caso recente com realização de biópsia muscular após 3 semanas de infecção leve por COVID-19 evidenciou uma redução da razão actina:miosina com perda dos filamentos de miosina, confirmando, portanto, a presença de miopatia primária pela COVID-19 como causa de fadiga crônica.(9) Estes achados abrem um nova perspectiva que sugere que a miopatia por lesão viral seja responsável pela persistência da fadiga na COVID-19 longa. Neste mesmo sentido, existe a hipótese de que estes pacientes possam desenvolver encefalomielite miálgica/síndrome de fadiga crônica pós-viral com possível neuropatia de fibras pequenas associada, como já descrito previamente nas infecções por outros vírus, ou no dano dos neurônios olfatórios, causando uma redução do fluxo do líquido cefalorraquidiano, com congestão do sistema glinfático e subsequente acúmulo tóxico no sistema nervoso central.(10,11)
A redução do V’O2 pico não foi o único achado descrito na síndrome pós-COVID-19. Alguns estudos relataram hiperventilação leve por aumento na ventilação minuto pela produção de CO2 (V’E/V’CO2) durante o esforço, que seria justificado por um aumento da quimiosensibilidade central(3) ou por respiração disfuncional,(5,6) o que reduziria a pressão arterial de CO2 por aumento do drive ventilatório. Deve-se considerar, entretanto, que estímulos sistêmicos da ventilação, como a ativação durante o exercício de ergorreceptores, como metabo- e mecanorreceptores, presentes nos músculos periféricos, também podem justificar o aumento da V’E/V’CO2 na ausência de sequelas pulmonares e cardíacas.(3) Outra possível causa para ineficiência ventilatória seria o aumento do espaço morto como fração do volume corrente (VEM/VC), que pode estar presente em pacientes com lesão endotelial e/ou microvascular, com pouca perfusão dos alvéolos, e/ou destruição do leito vascular pulmonar em áreas fibróticas, associado à redução da difusão de O2 pela barreira hemato-alveolar.(3,8) Tal hipótese seria plausível para justificar, por exemplo, a hipotética presença de hipertensão pulmonar de esforço (não confirmada até o momento na literatura).(3,8) Por fim, a relação VEM/VC também poderia estar elevada como consequência da redução do VC durante o exercício devido à persistência da fibrose intersticial pulmonar com consequente alteração da mecânica ventilatória e limitação ventilatória neste caso, com possível associação com a extensão do acometimento pulmonar intersticial agudo.(12) Por fim, a presença de hipoxemia induzida pelo esforço poderia levar à redução da oferta muscular de O2, causando limitação de trocas gasosas.(5)
Assim, à luz do conhecimento fisiopatológico atual de mecanismos de intolerância ao esforço e considerando-se a gama de manifestações sistêmicas da fase aguda da infecção por COVID-19, seria simplista de nossa parte considerar que todos os sintomas crônicos da síndrome da COVID-19 longa são decorrentes de descondicionamento físico por inatividade ou internação prolongada. A teoria do descondicionamento não explica a presença de sintomas persistentes em pacientes que foram acometidos por formas leves da doença, muitos dos quais nem sequer necessitaram de internação hospitalar. Da mesma forma, tal teoria não explica a dissociação entre a gravidade da internação hospitalar e a redução do V’O2 pico reportada até o momento, assim como não explica o antagonismo da persistência de sintomas em pacientes com V’O2 pico preservado.(4-6) Frente à potencialmente complexa e ainda desconhecida síndrome pós-COVID, é temeroso sermos simplistas na tentativa de desvendar seus mecanismos de intolerância ao exercício, é inaceitável sermos simplistas na tentativa de desvendar seus mecanismos de intolerância ao exercício. Precisamos de evidências científicas mais robustas antes de tirarmos conclusões reducionistas.
CONTRIBUIÇÕES DOS AUTORES EVMF e RKFO: elaboração, escrita e revisão do manuscrito.
REFERÊNCIAS 1. Nalbandian A, Sehgal K, Gupta A, et al. Post-acute COVID-19 syndrome. Nature Medicine 2021; 27: 601-615. https://doi.org/10.1038/s41591-021-01283-z.
2. Rinaldo RF, Mondoni M, Parazzini EM, et al. Severity does not impact on exercise capacity in COVID-19 survivors. Respiratory Medicine 2021;187:106577. https://doi.org/10.1016/j.rmed.2021.106577.
3. Singh I, Joseph P, Heerdt PM,et al. Persistent Exertional Intolerance After COVID-19: Insights From Invasive Cardiopulmonary Exercise Testing. CHEST 2021; S00123692(21)03635-7. https://doi.org/10.1016/j.chest.2021.08.010.
4. Rinaldo RF, Mondoni M, Parazzini EM, et al. Deconditioning as main mechanism of impaired exercise response in COVID-19 survivors. Eur Respir J 2021; 58: 2100870. https://doi.org/10.1183/ 13993003.00870-2021.
5. Skjørten I, Ankerstjerne OAW, Trebinjac D, et al. Cardiopulmonary exercise capacity and limitations 3 months after COVID-19 hospitalisation. Eur Respir J 2021; 58: 2100996. https://doi.org/10.1183/13993003.00996-2021.
6. Motiejunaite J, Balagny P, Arnoult F, et al. Hyperventilation as one of the mechanisms of persistent dyspnoea in SARS-CoV-2 survivors. Eur Respir J 2021; 58: 2101578. https://doi.org/10.1183/13993003.01578-2021.
7. Daniels CJ, Rajpal S, Greenshields JT, et al. Prevalence of Clinical and Subclinical Myocarditis in Competitive Athletes With Recent SARS-CoV-2 Infection Results From the Big Ten COVID-19 Cardiac Registry. JAMA Cardiol. 2021;6(9):1078-1087. https://doi.org/10.1001/jamacardio.2021.2065.
8. Baratto C, Caravita S, Faini A, et al. Impact of COVID-19 on exercise pathophysiology: a combined cardiopulmonary and echocardiographic exercise study. J Appl Physiol 2021; 130: 1470-1478. https://doi.org/10.1152/japplphysiol.00710.2020.
9. Belén Rodriguez, S. Nansoz, D.R. Cameron et al. Is myopathy part of long-Covid? Clinical Neurophysiology 2021;132:1241-1242. https://doi.org/10.1016/j.clinph.2021.03.008.
10. Wostyn, P. COVID-19 and chronic fatigue syndrome: Is the worst yet to come? Medical Hypotheses 2021; 146:110469. https://doi.org/10.1016/j.mehy.2020.110469.
11. Joseph, P, Arevalo, C, Oliveira RFK, et al. Insights From Invasive Cardiopulmonary Exercise Testing of Patients With Myalgic Encephalomyelitis/Chronic Fatigue Syndrome. Chest 2021; 160(2):642-651. https://doi.org/10.1016/j.chest.2021.01.082.
12. Liu M, Lv F, Huang Y and Xiao K. Follow-Up Study of the Chest CT Characteristics of COVID-19 Survivors Seven Months After Recovery. Front. Med. 2021. 8:636298. https://doi.org/10.3389/fmed.2021.636298.