AO EDITOR,Disfunção endotelial e comprometimento muscular periférico são conhecidas manifestações extrapulmonares da DPOC.(1,2) Propôs-se que a quantificação de vesículas extracelulares (VE) derivadas de células endoteliais (VEE) é um método possível para avaliar a função endotelial.(3) Além disso, VEE podem ser marcadores de enfisema e preditores de prognóstico na DPOC.(4) Embora atualmente se recomende atividade física para pacientes com DPOC,(2) não se sabe que efeito uma única sessão de exercício físico tem na resposta endotelial nesses pacientes. Propôs-se que a função vascular de indivíduos saudáveis após uma sessão aguda de exercício físico segue um padrão bifásico (uma diminuição transitória seguida de normalização ou até mesmo de melhoria).(5) No entanto, isso ainda não foi estudado em pacientes com DPOC. Realizamos um estudo piloto para avaliar VEE (como possíveis marcadores de resposta endotelial) após exercício agudo em pacientes com DPOC. Nossa hipótese foi a de que haveria um aumento de VEE após o exercício agudo.
Foram incluídos pacientes com diagnóstico confirmado de DPOC baseado em diretrizes atuais,(2) VEF1 pós-broncodilatador entre 30% e 70% do valor previsto e sem comorbidades graves. Indivíduos saudáveis formaram o grupo controle. O estudo foi aprovado pelo comitê de ética em pesquisa da instituição, em conformidade com a Declaração de Helsinque, e todos os participantes assinaram um termo de consentimento livre e esclarecido. Após a avaliação clínica, todos os participantes foram submetidos a exames bioquímicos de sangue e testes de função pulmonar (visita 0). Na visita 1, todos os participantes foram submetidos a um teste de exercício cardiopulmonar (TECP) incremental limitado por sintomas em um cicloergômetro, usando um protocolo incremental em rampa baseado em sua função pulmonar basal para concluir o teste em 6-10 min. Durante o TECP, foram coletados dados sobre variáveis metabólicas, respiratórias e hemodinâmicas centrais (Tabela 1). Na visita 2, os participantes realizaram um TECP em carga constante após um período de aquecimento de 3 min e com 70% da carga máxima atingida durante o teste incremental. Para medir as VE plasmáticas, foram coletadas amostras de sangue periférico em repouso (T0), no pico do exercício (Tmáx, isto é, assim que os participantes pararam de pedalar, por exaustão) e na recuperação (Trec, isto é, 1 h depois da conclusão do TECP). Tanto o TECP incremental como o TECP em velocidade constante foram realizados pela manhã, aproximadamente 2 h após um café da manhã leve (sem café), com 5-7 dias de intervalo. Pedimos aos fumantes que não fumassem antes de cada visita. O plasma pobre em plaquetas foi obtido por meio de duas centrifugações (1.500 g por 15 min e 13.000 g por 2 min). Para a caracterização das VE plasmáticas, foi realizada a citometria de fluxo multiparamétrica, com um citômetro de fluxo BD FACS Canto (BD Biosciences, San Jose, CA, EUA), como se descreveu anteriormente,(6) porém com algumas modificações. As VEE foram inicialmente discriminadas por tamanho como eventos (em conformidade com uma distribuição de dispersão de luz dentro da faixa de esferas de 0,5 a 0,9 µm) e posteriormente definidas como eventos positivos para anti-anexina V marcada com complexo peridinina-clorofila-proteína, éster succinimidil de diacetato de carboxifluoresceína, anti-CD62e marcado com aloficocianina e anti-CD31 marcado com ficoeritrina
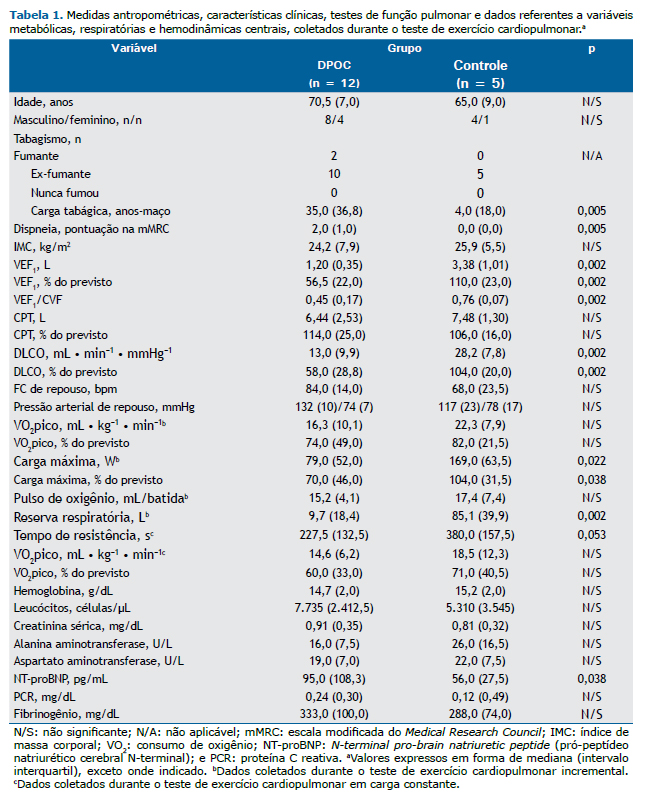
Os dados são apresentados em forma de mediana e intervalo interquartil. Foram usados o teste de Kolmogorov-Smirnov para duas amostras e o teste de Friedman para medidas repetidas. Todas as análises estatísticas foram realizadas com o programa IBM SPSS Statistics, versão 20.0 (IBM Corporation, Armonk, NY, EUA).
Foram incluídos no estudo 17 indivíduos: 12 pacientes com DPOC (grupo DPOC) e 5 indivíduos saudáveis (grupo controle). Os dois grupos não diferiram nas medidas antropométricas. O grupo DPOC apresentou obstrução moderada do fluxo aéreo e diminuição moderada da DLCO na espirometria (Tabela 1). Houve diferença entre os dois grupos quanto à resposta de VEE. Não foram encontradas diferenças no grupo controle quanto aos níveis de VEE em T0, Tmáx e Trec: 11,8 (37,6) eventos/min, 14,8 (31,1) eventos/min e 19,6 (30,1) eventos/min, respectivamente (p = 0,678). No grupo DPOC, os níveis de VEE foram significativamente menores em Tmáx do que em T0 [3,4 (14,1) eventos/min vs. 6,7 (16,9) eventos/min, p = 0,024] e Trec [3,4 (14,1) eventos/min vs. 7,8 (35,1) eventos/min, p = 0,002], sem diferença entre os níveis de VEE em T0 e Trec. Atribuímos arbitrariamente a T0 um nível de VEE de 100% e calculamos os níveis de VEE em Tmáx e Trec, bem como variações relativas da linha de base. Não foram encontradas diferenças no grupo controle. No entanto, no grupo DPOC, os níveis de VEE foram significativamente menores em Tmáx do que em T0 [62,3% (27,6%) vs. 100,0% (0,0%), p = 0,024] e Trec [62,3% (27,6%) vs. 109,5% (156,7%), p = 0,002]. Essa diminuição dos níveis de VEE em Tmáx, com retorno aos níveis basais em Trec, foi observada em 11 dos 12 pacientes com DPOC.
Pelo que sabemos, nenhum estudo investigou o comportamento de VEE circulantes após o exercício em pacientes com DPOC. Embora se espere que os níveis circulantes de VE aumentem em indivíduos saudáveis, os resultados variam no que tange à origem celular das VE e aos níveis de VE após o exercício.(7) Um estudo(8) mostrou um aumento dos níveis circulantes de VEE em uma amostra composta por 17 pacientes com DPOC; entretanto, é difícil comparar esses resultados com os nossos porque esse aumento ocorreu após estresse de cisalhamento induzido experimentalmente, e não após o exercício. Os níveis reduzidos de VEE circulantes após o exercício parecem ser característicos da fisiopatologia da DPOC e podem representar uma depuração exagerada de VEE no pico do exercício nesses pacientes. Em atletas, observou-se que o exercício físico protege o sistema vascular e promove uma captação in vitro de VEE em células endoteliais que está relacionada com proteção de células-alvo contra a apoptose.(9) De modo semelhante, é possível que o exercício agudo possa causar ativação endotelial (protetora ou prejudicial) apenas em pacientes com DPOC: é possível que a redução de VEE observada por nós tenha resultado da depuração exagerada de VEE pelo endotélio; como a patogênese da DPOC envolve o leito capilar pulmonar,(10) podemos especular sobre a ativação de um endotélio pulmonar alterado especificamente durante o exercício nesses pacientes (ao passo que nos indivíduos do grupo controle, nos quais se espera que a função endotelial pulmonar seja normal, não observamos redução significativa de VEE). É preciso investigar a fundo se esse fenômeno realmente envolve ou não o leito vascular pulmonar usando o fator de von Willebrand como marcador de células endoteliais para determinar a origem das VE.
CONTRIBUIÇÕES DOS AUTORESDN, TN, e AC: concepção e desenho do estudo; DN, TN, SS, e SL: testes e análises laboratoriais; DN, TN, e AC: análise de dados; DN, TN, e AC: preparação do manuscrito; e DN, TN, SS, SL, e AC: revisão e aprovação final do manuscrito.
REFERÊNCIAS1. Schivo M, Albertson TE, Haczku A, Kenyon NJ, Zeki AA, Kuhn BT, et al. Paradigms in chronic obstructive pulmonary disease: phenotypes, immunobiology, and therapy with a focus on vascular disease. J Investig Med. 2017;65(6):953-963. https://doi.org/10.1136/jim-2016-000358
2. Vogelmeier CF, Criner GJ, Martinez FJ, Anzueto A, Barnes PJ, Bourbeau J, et al. Global Strategy for the Diagnosis, Management, and Prevention of Chronic Obstructive Lung Disease 2017 Report. GOLD Executive Summary. Am J Respir Crit Care Med. 2017;195(5):557-582. https://doi.org/10.1164/rccm.201701-0218PP
3. Lekakis J, Abraham P, Balbarini A, Blann A, Boulanger CM, Cockcroft J, et al. Methods for evaluating endothelial function: a position statement from the European Society of Cardiology Working Group on Peripheral Circulation. Eur J Cardiovasc Prev Rehabil. 2011;18(6):775-789. https://doi.org/10.1177/1741826711398179
4. Nieri D, Neri T, Petrini S, Vagaggini B, Paggiaro P, Celi A. Cell-derived microparticles and the lung. Eur Respir Rev. 2016;25(141):266-277. https://doi.org/10.1183/16000617.0009-2016
5. Dawson EA, Green DJ, Cable NT, Thijssen DH. Effects of acute exercise on flow-mediated dilatation in healthy humans. J Appl Physiol (1985). 2013;115(11):1589-1598. https://doi.org/10.1152/japplphysiol.00450.2013
6. Neri T, Tavanti L, De Magistris S, Lombardi S, Romei C, Falaschi F, Paggiaro P, Celi A. Endothelial Cell-Derived Extracellular Vesicles as Potential Biomarkers in Chronic Interstitial Lung Diseases. Ann Clin Lab Sci. 2019;49(5):608-610. https://doi.org/10.1183/13993003.congress-2019.PA2253
7. Highton PJ, Martin N, Smith AC, Burton JO, Bishop NC. Microparticles and Exercise in Clinical Populations. Exerc Immunol Rev. 2018;24:46-58.
8. Barak OF, Mladinov S, Hoiland RL, Tremblay JC, Thom SR, Yang M, et al. Disturbed blood flow worsens endothelial dysfunction in moderate-severe chronic obstructive pulmonary disease. Sci Rep. 2017;7(1):16929. https://doi.org/10.1038/s41598-017-17249-6
9. Wahl P, Jansen F, Achtzehn S, Schmitz T, Bloch W, Mester J, et al. Effects of high intensity training and high volume training on endothelial microparticles and angiogenic growth factors. PLoS One. 2014;9(4):e96024. https://doi.org/10.1371/journal.pone.0096024
10. Kanazawa H, Asai K, Hirata K, Yoshikawa J. Possible effects of vascular endothelial growth factor in the pathogenesis of chronic obstructive pulmonary disease. Am J Med. 2003;114(5):354-358. https://doi.org/10.1016/S0002-9343(02)01562-0