ABSTRACT
Objective: To identify the characteristics of thoracic calcifications on magnetic resonance (MR) imaging, as well as correlations between MR imaging and CT findings. Methods: This was a retrospective study including data on 62 patients undergoing CT scans and MR imaging of the chest at any of seven hospitals in the Brazilian states of Rio Grande do Sul, São Paulo, and Rio de Janeiro between March of 2014 and June of 2016 and presenting with calcifications on CT scans. T1- and T2-weighted MR images (T1- and T2-WIs) were semiquantitatively analyzed, and the lesion-to-muscle signal intensity ratio (LMSIR) was estimated. Differences between neoplastic and non-neoplastic lesions were analyzed. Results: Eighty-four calcified lesions were analyzed. Mean lesion density on CT was 367 ± 435 HU. Median LMSIRs on T1- and T2-WIs were 0.4 (interquartile range [IQR], 0.1-0.7) and 0.2 (IQR, 0.0-0.7), respectively. Most of the lesions were hypointense on T1- and T2-WIs (n = 52 [61.9%] and n = 39 [46.4%], respectively). In addition, 19 (22.6%) were undetectable on T1-WIs (LMSIR = 0) and 36 (42.9%) were undetectable on T2-WIs (LMSIR = 0). Finally, 15.5% were hyperintense on T1-WIs and 9.5% were hyperintense on T2-WIs. Median LMSIR was significantly higher for neoplastic lesions than for non-neoplastic lesions. There was a very weak and statistically insignificant negative correlation between lesion density on CT and the following variables: signal intensity on T1-WIs, LMSIR on T1-WIs, and signal intensity on T2-WIs (r = −0.13, p = 0.24; r = −0.18, p = 0.10; and r = −0.16, p = 0.16, respectively). Lesion density on CT was weakly but significantly correlated with LMSIR on T2-WIs (r = −0.29, p < 0.05). Conclusions: Thoracic calcifications have variable signal intensity on T1- and T2-weighted MR images, sometimes appearing hyperintense. Lesion density on CT appears to correlate negatively with lesion signal intensity on MR images.
Keywords:
Calcification, physiologic; Thorax/diagnostic imaging; Tomography, X-ray computed; Magnetic resonance imaging.
RESUMO
Objetivo: Identificar as características das calcificações torácicas na ressonância magnética (RM) e as correlações entre os achados de RM e TC. Métodos: Estudo retrospectivo no qual foram analisados dados referentes a 62 pacientes que foram submetidos a TC e RM de tórax em sete hospitais nos estados do Rio Grande do Sul, São Paulo e Rio de Janeiro entre março de 2014 e junho de 2016 e que apresentaram calcificações na TC. As imagens de RM ponderadas em T1 e T2 (doravante denominadas T1 e T2) foram analisadas semiquantitativamente, e a razão entre a intensidade do sinal da lesão e do músculo (LMSIR, do inglês lesion-to-muscle signal intensity ratio) foi estimada. Diferenças entre lesões neoplásicas e não neoplásicas foram analisadas. Resultados: Foram analisadas 84 lesões calcificadas. A média de densidade das lesões na TC foi de 367 ± 435 UH. A mediana da LMSIR foi de 0,4 [intervalo interquartil (II): 0,1-0,7] em T1 e 0,2 (II: 0,0-0,7) em T2. A maioria das lesões mostrou-se hipointensa em T1 e T2 [n = 52 (61,9%) e n = 39 (46,4%), respectivamente]. Além disso, 19 (22,6%) foram indetectáveis em T1 (LMSIR = 0) e 36 (42,9%) foram indetectáveis em T2 (LMSIR = 0). Finalmente, 15,5% mostraram-se hiperintensas em T1 e 9,5% mostraram-se hiperintensas em T2. A mediana da LMSIR foi significativamente maior nas lesões neoplásicas do que nas não neoplásicas. Houve uma correlação negativa muito fraca e estatisticamente insignificante entre a densidade das lesões na TC e as seguintes variáveis: intensidade do sinal em T1, LMSIR em T1 e intensidade do sinal em T2 (r = −0,13, p = 0,24; r = −0,18, p = 0,10 e r = −0,16, p = 0,16, respectivamente). A densidade das lesões na TC apresentou correlação fraca, porém significativa com a LMSIR em T2 (r = −0,29, p < 0,05). Conclusões: As calcificações torácicas apresentam intensidade de sinal variável em T1 e T2; em alguns casos, mostram-se hiperintensas. A densidade da lesão na TC aparentemente correlaciona-se negativamente com a intensidade do sinal da lesão na RM.
Palavras-chave:
Calcificação fisiológica; Tórax/diagnóstico por imagem; Tomografia computadorizada por raios X; Imagem por ressonância magnética.
INTRODUÇÃOAs calcificações torácicas relacionam-se com diversas doenças, tais como granulomas calcificados, doenças metabólicas, doenças ocupacionais e metástases pulmonares, bem como com tumores benignos e malignos.(1-5) A TC é o método padrão ouro para detectar e caracterizar calcificações.(1) Há poucos estudos sobre como as calcificações torácicas aparecem na ressonância magnética (RM); a maioria dos estudos sobre calcificações na RM envolve lesões intracranianas.(6-10)
As calcificações geram um sinal de intensidade inespecífica em imagens ponderadas em T1 e T2 (doravante denominadas T1 e T2) e em imagens gradiente-eco.(7,8,11) Como não contêm prótons móveis, os sais de cálcio não apresentam sinal na RM, e a descrição clássica da intensidade do sinal de lesões muito calcificadas em T1 e T2 é a de que é baixa.(6-8,11) No entanto, há relatos de sinais hiperintensos, hipointensos e isointensos em T1 e T2; a intensidade do sinal depende da composição específica dos agregados de sais de cálcio e do tamanho das partículas.(7,8,11,12)
Embora a radiografia e a TC tenham sido amplamente usadas na avaliação torácica, a RM de tórax é uma modalidade nova.(13-17) Como combina imagens funcionais e morfológicas, a RM de tórax é uma alternativa para pacientes nos quais a exposição à radiação ionizante é uma preocupação (crianças, gestantes e pacientes submetidos à RM repetidas vezes durante um longo período, por exemplo).(13,14) O principal objetivo do presente estudo foi identificar as características das calcificações torácicas na RM e as correlações entre os achados de RM e TC. O objetivo secundário foi comparar as calcificações relacionadas com câncer e as não relacionadas com câncer quanto às suas características na RM.
MÉTODOSParticipantesTrata-se de um estudo retrospectivo no qual foram analisados dados referentes a pacientes submetidos a TC e RM de tórax em sete hospitais nos estados do Rio Grande do Sul, São Paulo e Rio de Janeiro entre março de 2014 e junho de 2016. O comitê de ética em pesquisa da instituição aprovou o estudo (Protocolo n. 22758413.8.0000.5335) e dispensou o consentimento livre e esclarecido.
Os critérios de inclusão foram os seguintes: calcificações torácicas > 0,3 cm na TC de tórax e RM de tórax com qualidade diagnóstica. Todos os pacientes que compuseram nossa amostra haviam participado de estudos prévios nos quais se comparou o uso de TC e RM na avaliação de nódulos e vasos pulmonares e no estadiamento do câncer de pulmão. Tanto a RM de tórax como a TC de tórax foram realizadas na mesma semana. Os prontuários médicos dos pacientes foram analisados, e as lesões pulmonares foram classificadas em neoplásicas e não neoplásicas.
Protocolos de RM e TCA TC foi realizada com um tomógrafo multidetector de 64 canais (LightSpeed VCT; GE Healthcare, Chicago, IL, EUA). Foram usados os seguintes parâmetros: 120 kVp; 250 mA; tempo de rotação = 0,8 s; pitch = 1,375. A aquisição volumétrica inspiratória foi realizada com 1 mm de colimação em incrementos de 1 mm e um algoritmo de reconstrução de tecidos moles. A TC foi realizada com janela para o mediastino (largura: 350-450 UH; nível: 20-40 UH) e o parênquima pulmonar (largura: 1.200-1.600 UH; nível: −500 UH a −700 UH), e as reconstruções foram realizadas no plano axial e coronal.
A RM foi realizada em um aparelho de 1,5 T (Magnetom Aera; Siemens Healthineers, Erlangen, Alemanha). Para a recepção do sinal, foi usado um sistema especial de bobinas matrizes integradas com 12 elementos cobrindo todo o tórax.(18) O sistema consiste em duas bobinas flexíveis de sinergia (uma anterior e outra posterior), cada qual com seis elementos receptores. (18) Foi usada uma sequência HASTE, e o campo de visão (field of view) foi adaptado a cada paciente. A sequência HASTE foi realizada com gating respiratório e navegador diafragmático. Os parâmetros da sequência foram os seguintes: tempo de repetição (TR)/tempo de eco (TE)/ângulo de inclinação (flip angle) = infinito/92 ms/150°; fator de aquisição paralela = 2; espessura de corte = 5 mm; distância (distance factor) = 20%; orientação transversal e coronal (matrizes de 380 × 256 e 400 × 320, respectivamente); tempo de aquisição = aproximadamente 90 s. Uma sequência VIBE foi usada para obter imagens rápidas ponderadas em T1. Os parâmetros da sequência tridimensional foram os seguintes: TR/TE = 5,12 ms/2,51 ms, ângulo de inclinação = 10°, espessura de corte = 5 mm (sem intervalo entre os cortes) e matriz de 256 × 116, com pausa respiratória. Também foi usada uma sequência PROPELLER ponderada em T2 com saturação de gordura (BLADE; Siemens Healthineers), cujos parâmetros foram os seguintes: TR/TE = 4.670 ms/113 ms e espessura de corte = 5 mm, sem intervalo entre os cortes. A difusão (diffusion-weighted imaging) foi realizada por meio da técnica ecoplanar de disparo único, com espessura de corte = 6 mm, recuperação de inversão atenuada espectral e escaneamento acionado pela respiração. Os parâmetros da sequência de difusão foram os seguintes: TR/TE/ângulo de inclinação = 3.000-4.500 ms/65 ms/90°; gradiente de difusão em três planos ortogonais; b = 50, 400 e 800 s/mm2; campo de visão = 350 mm e matriz de 128 × 128. A média do tempo de permanência na sala de RM foi de aproximadamente 15 min, e não houve necessidade de sedação.
Análise das imagensAs imagens de TC e RM foram analisadas independentemente por dois radiologistas torácicos que tinham mais de 7 anos de experiência e que desconheciam as informações clínicas a respeito dos pacientes. Em seguida, os dois radiologistas juntos analisaram as imagens a fim de tomar uma decisão consensual final. Os critérios para os achados de TC e RM foram os definidos no Glossário de Termos da Sociedade Fleischner.(19)
Durante a análise tomográfica, os radiologistas torácicos identificaram áreas de calcificação em janela mediastinal, medindo a média de densidade em uma região de interesse (ROI, do inglês region of interest) que incluía pelo menos 90% da calcificação identificada na TC. Em seguida, as calcificações foram classificadas em difusas (áreas difusas ou segmentadas de calcificação óssea), puntiformes (áreas micronodulares de calcificação óssea) e laminares (áreas lineares de calcificação óssea). Todas as imagens foram analisadas em uma estação de trabalho especial (Advantage Workstation 4.2; GE Healthcare), com um sistema de arquivamento e comunicação de imagens.
As imagens de RM foram analisadas semiquantitativamente pelos radiologistas supracitados, que definiram manu-almente ROI tridimensionais através da composição dos limites bidimensionais das lesões traçados em cortes conse-cutivos; as calcificações observadas na TC foram usadas como referência. Além disso, uma ROI foi traçada no mús-culo paravertebral no mesmo nível em T1 e T2 axiais (média de área: 50-80 mm2, isto é, 14-30 pixels), e a razão entre a intensidade do sinal da lesão e do músculo (LMSIR, do inglês lesion-to-muscle signal intensity ratio) foi esti-mada. A LMSIR < 1 caracterizou lesão hipointensa, a LMSIR = 1 caracterizou lesão isointensa, a LMSIR > 1 caracte-rizou lesão hiperintensa e a LMSIR = 0 caracterizou lesão indetectável por RM (efeito nulo).
Análise estatísticaTodas as análises estatísticas foram realizadas por meio do programa Statistical Package for the Social Sciences, versão 11.0 (SPSS Inc., Chicago, IL, EUA). As variáveis contínuas foram expressas em forma de média ± desvio-padrão ou mediana e intervalo interquartil (II), ao passo que as variáveis categóricas foram expressas em forma de números absolutos e proporções. As variáveis contínuas com distribuição normal foram comparadas pelo teste t de Student para amostras independentes, ao passo que as variáveis contínuas sem distribuição normal foram comparadas pelo teste U de Mann-Whitney. As variáveis categóricas foram comparadas pelo teste exato de Fisher. O nível de significância adotado foi de p < 0,05.
Coeficientes de correlação de Pearson foram usados para avaliar correlações entre variáveis contínuas: coeficientes = 0,00-0,20 indicaram correlação muito fraca; coeficientes ≥ 0,20-0,40 indicaram correlação fraca; coeficientes ≥ 0,40-0,70 indicaram correlação moderada; coeficientes ≥ 0,70-0,90 indicaram correlação forte; coeficientes ≥ 0,90 indicaram correlação muito forte.(20)
RESULTADOSA amostra estudada foi composta por 62 pacientes (84 lesões calcificadas). Dos 62 pacientes, 37 (59,7%) eram do sexo feminino. A média de tamanho das ROI foi de 22 mm2 (8-49 mm2). Das 84 lesões, 46 (54,8%) eram solitárias. Dos 62 pacientes que compuseram a amostra estudada, 36 haviam participado de um estudo no qual se comparou o uso de TC e RM na avaliação de nódulos pulmonares, 18 haviam participado de um estudo no qual se comparou o uso de TC e RM na avaliação de vasos pulmonares e 8 haviam participado de um estudo no qual se comparou o uso de TC e RM no estadiamento do câncer de pulmão.
A média de densidade das lesões na TC foi de 367 ± 435 UH. Das 84 lesões analisadas, 56 (66,7%) eram lesões pulmonares, 5 (5,9%) eram lesões pleurais e 23 (27,4%) eram lesões mediastinais. Além disso, 65 (77,4%) eram difusas, 15 (17,8%) eram laminares e 4 (4,8%) eram puntiformes. A mediana da LMSIR foi de 0,4 (II: 0,1-0,7) em T1 e 0,2 (II: 0,0-0,7) em T2.
A maioria das lesões mostrou-se hipointensa em T1 e T2 [n = 52 (61,9%) e n = 39 (46,4%), respectivamente]. Além disso, 19 (22,6%) foram indetectáveis em T1 (LMSIR = 0) e 36 (42,9%) foram indetectáveis em T2 (LMSIR = 0). Finalmente, 15,5% mostraram-se hiperintensas em T1 e 9,5% mostraram-se hiperintensas em T2 (Figura 1).
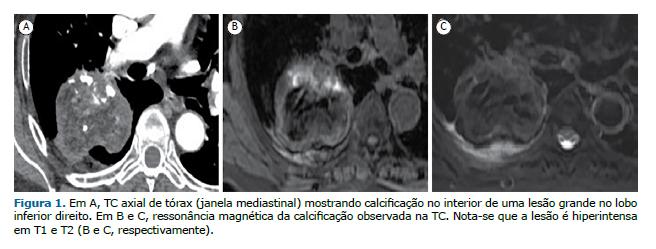
Como se pode observar na Tabela 1, houve diferença significativa entre as lesões neoplásicas e não neoplásicas quanto ao diâmetro mínimo e ao diâmetro máximo (p < 0,05). Embora as lesões neoplásicas e não neoplásicas tenham se mostrado hipointensas em T1 e T2, a mediana da LMSIR foi significativamente maior nas lesões neoplásicas do que nas não neoplásicas (Tabela 1). Embora todas as lesões neoplásicas tenham sido detectáveis por RM (Figuras 1 e 2), 25% e 47,4% das lesões não neoplásicas foram indetectáveis em T1 e T2, respectivamente (Tabela 1).
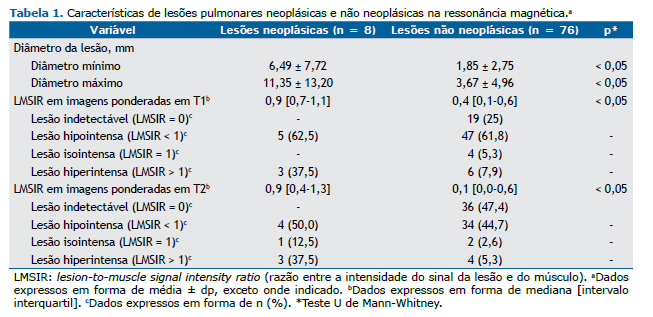
A Tabela 2 mostra a LMSIR em T1 e T2, de acordo com o tipo de calcificação. A maioria das calcificações difusas mostrou-se hipointensa ou foi indetectável em T1 e T2. A maioria das calcificações laminares mostrou-se hipointensa, e todas apresentaram intensidade de sinal em T1 e T2.
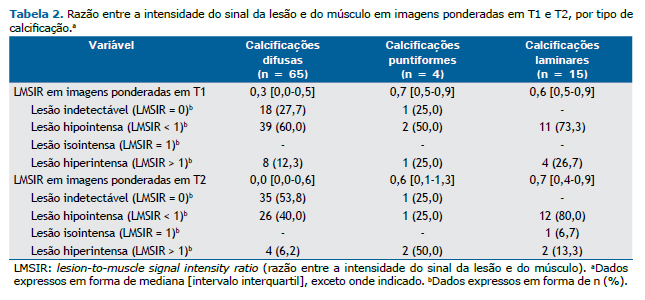
Houve uma correlação negativa muito fraca e estatisticamente insignificante entre a densidade das lesões na TC e as seguintes variáveis: intensidade do sinal em T1, LMSIR em T1 e intensidade do sinal em T2 (r = −0,13, p = 0,24; r = −0,18, p = 0,10 e r = −0,16, p = 0,16, respectivamente). Além disso, a densidade das lesões na TC apresentou correlação fraca, porém significativa com a LMSIR em T2 (r = −0,29, p < 0,05).
DISCUSSÃOO presente estudo mostrou que as calcificações torácicas apresentam intensidade de sinal variável em T1 e T2. A composição química das calcificações inclui fosfato de cálcio cristalino e hidroxiapatita, além de uma pequena quantidade de cobre, manganês, zinco, magnésio e ferro.(8) Como as concentrações em diferentes calcificações fisiológicas e patológicas variam, sua aparência na RM é heterogênea.(8) Essa variação na aparência ocorre em virtude de um mecanismo de relaxamento de superfície, que reduz os tempos de relaxamento T1 e T2.(6) Materiais que têm o mesmo tamanho e composição química podem ter efeitos nitidamente diferentes no relaxamento, dependendo do grau de irregularidade da superfície. (6,8) As propriedades inerentes do cálcio podem causar hiperintensidade em T1. O efeito T1 é predominante em casos em que a superfície é muito irregular e sua área é muito grande, como é o caso dos cristais de cálcio, aumentando a intensidade do sinal de RM.(6) Esse pode ser o motivo pelo qual a proporção de lesões hiperintensas foi maior em T1 que em T2 no presente estudo.
À medida que a concentração de cálcio aumenta para mais de 30-40%, a densidade de prótons diminui, o que resulta em diminuição progressiva da intensidade do sinal.(6) Lesões calcificadas mostram-se hipointensas na RM em virtude da diminuição da densidade de prótons. (6-10) No presente estudo, observou-se uma correlação negativa, porém estatisticamente insignificante entre a densidade das lesões na TC e a intensidade do sinal das lesões na RM, o que corrobora o achado de que lesões com maior concentração de cálcio tendem a apresentar menor intensidade de sinal na RM. Isso também explicaria por que a mediana da LMSIR em T1 e T2 foi menor nas calcificações difusas do que nas calcificações puntiformes e laminares.
Estudos anteriores descreveram calcificações neoplásicas hiperintensas na RM.(7,8,10,21) No presente estudo, as calcificações neoplásicas mostraram-se variáveis na RM. No entanto, a mediana da LMSIR foi significativamente maior em lesões hiperintensas do que em lesões hipointensas (37,5% vs. 7,9% em T1 e 37,5% vs. 5,3% em T2), o que pode ser atribuído à alteração dos efeitos de superfície que partículas diamagnéticas causam no sinal de RM e à consequente redução da T1 de prótons de água em virtude de baixas concentrações de cálcio.
Nosso estudo tem limitações, algumas das quais são inerentes a sua natureza retrospectiva. São necessários estudos maiores, prospectivos, para confirmar os achados de nossa análise de subgrupos. Além disso, estudos futuros devem incluir outras sequências além das spin-eco ponderadas em T1 e T2 convencionais.
Os desafios técnicos para o sucesso da RM do pulmão incluem a baixa densidade do tecido (que resulta em diminuição da intensidade do sinal) e diferenças de suscetibilidade magnética entre o tecido e o ar.(22) Alguns estudos empregaram a RM em sequências gradiente-eco e o mapeamento quantitativo de suscetibilidade magnética para caracterizar lesões cerebrais calcificadas.(7) A sequência de suscetibilidade magnética (SWI, do inglês susceptibility-weighted imaging) é útil para diferenciar calcificações intracranianas de hemorragias intracranianas, cuja atenuação pode ser semelhante na TC.(7) Pelo que sabemos, entretanto, ainda não há estudos sobre o uso da SWI na RM de tórax.
Outra limitação de nosso estudo é o uso de uma média de intensidade de sinal em uma ROI pré-definida. A intensidade do sinal de uma lesão calcificada varia; é possível, por exemplo, que a periferia seja hiperintensa e a intensidade diminua em direção ao centro.(6) No entanto, acreditamos que isso tenha pouco impacto na prática clínica.
Em suma, as calcificações torácicas (particularmente as calcificações neoplásicas) apresentam intensidade de sinal variável em T1 e T2, e a densidade da lesão na TC aparentemente correlaciona-se negativamente com a intensidade do sinal da lesão na RM. Os radiologistas devem estar cientes desses achados ao interpretar imagens de RM de tórax.
AGRADECIMENTOSGostaríamos de agradecer ao Professor Hans Ulrich Kauczor suas sugestões.
REFERÊNCIAS1. Bendayan D, Barziv Y, Kramer MR. Pulmonary calcifications: a review. Respir Med. 2000;94(3):190-3. https://doi.org/10.1053/rmed.1999.0716
2. Winer-Muram HT. The solitary pulmonary nodule. Radiology. 2006;239(1):34-49. https://doi.org/10.1148/radiol.2391050343
3. Khan AN, Al-Jahdali HH, Allen CM, Irion KL, Al-Ghanem S, Koteyar SS. The calcified lung nodule: What does it mean? Ann Thorac Med. 2010;5(2):67-79. https://doi.org/10.4103/1817-1737.62469
4. Ceylan N, Bayraktaroglu S, Savas R, Alper H. CT findings of high-attenuation pulmonary abnormalities. Insights Imaging. 2010;1(4):287-292. https://doi.org/10.1007/s13244-010-0039-2
5. Taguchi Y, Fuyuno G, Shioya S, Yanagimachi N, Katoh H, Matsuyama S, et al. MR appearance of pulmonary metastatic calcification. J Comput Assist Tomogr. 1996;20(1):38-41. https://doi.org/10.1097/00004728-199601000-00008
6. Henkelman RM, Watts JF, Kucharczyk W. High signal intensity in RM images of calcified brain tissue. Radiology. 1991;179(1):199-206. https://doi.org/10.1148/radiology.179.1.1848714
7. Chen W, Zhu W, Kovanlikaya I, Kovanlikaya A, Liu T, Wang S, et al. Intracranial calcifications and hemorrhages: characterization with quantitative susceptibility mapping. Radiology. 2014;270(2):496-505. https://doi.org/10.1148/radiol.13122640
8. Wu Z, Mittal S, Kish K, Yu Y, Hu J, Haacke EM. Identification of calcification with MRI using susceptibility-weighted imaging: a case study. J Magn Reson Imaging. 2009;29(1):177-82. https://doi.org/10.1002/jmri.21617
9. Iglesia MM, Cortés GL, Icaran DG, Cortes IL, Oleaga L, Isusi M. Calcificaciones intracraneales: Imagen por RM. Radiología. 2006;48(1):19-26. https://doi.org/10.1016/S0033-8338(06)73125-3
10. Hochhegger B, Marchiori E, Soares Souza A Jr, Soares Souza L, Palermo L. MRI and CT findings of metastatic pulmonary calcification. Br J Radiol. 2012;85(1011):e69-72. https://doi.org/10.1259/bjr/53649455
11. Gamsu G, De Geer G, Cann C, Muller N, Brito A. A preliminary study of MRI quantification of simulated calcified pulmonary nodules. Invest Radiol. 1987;22(11):853-8. https://doi.org/10.1097/00004424-198711000-00001
12. Yi CA, Jeon TY, Lee KS, Lee JH, Seo JB, Kim YK, et al. 3-T MRI: usefulness for evaluating primary lung cancer and small nodules in lobes not containing primary tumors. AJR Am J Roentgenol. 2007;189(2):386-92. https://doi.org/10.2214/AJR.07.2082
13. Wild JM, Marshall H, Bock M, Schad LR, Jakob PM, Puderbach M, et al. MRI of the lung (1/3): methods. Insights Imaging. 2012;3(4):345-53. https://doi.org/10.1007/s13244-012-0176-x
14. Biederer J, Beer M, Hirsch W, Wild J, Fabel M, Puderbach M, et al. MRI of the lung (2/3). Why .when . how? Insights Imaging. 2012;3(4):355-71. https://doi.org/10.1007/s13244-011-0146-8
15. Barreto MM, Rafful PP, Rodrigues RS, Zanetti G, Hochhegger B, Souza AS Jr, et al. Correlation between computed tomographic and magnetic resonance imaging findings of parenchymal lung diseases. Eur J Radiol. 2013;82(9):e492-501. https://doi.org/10.1016/j.ejrad.2013.04.037
16. Pasin L, Zanon M, Moreira J, Moreira AL, Watte G, Marchiori E, et al. Magnetic Resonance Imaging of Pulmonary Embolism: Diagnostic Accuracy of Unenhanced MR and Influence in Mortality Rates. Lung. 2017;195(2):193-199. https://doi.org/10.1007/s00408-017-9975-7
17. Weinreb JC. Which study when? Is Gadolinium-enhanced MR imaging safer than iodine-enhanced CT? Radiology. 2008;249(1):3-8. https://doi.org/10.1148/radiol.2491080075
18. Maglione M, Montella S, Mollica C, Carnovale V, Iacotucci P, De Gregorio F, et al. Lung structure and function similarities between primary ciliary dyskinesia and mild cystic fibrosis: a pilot study. Ital J Pediatr. 2017;43(1):34. https://doi.org/10.1186/s13052-017-0351-2
19. Hansell DM, Bankier AA, MacMahon H, McLoud TC, Müller NL, Remy J. Fleischner Society: glossary of terms for thoracic imaging. Radiology. 2008;246(3):697-722. https://doi.org/10.1148/radiol.2462070712
20. Mukaka MM. Statistics corner: A guide to appropriate use of correlation coefficient in medical research. Malawi Med J 2012;24(3):69-71.
21. Henz Concatto N, Watte G, Marchiori E, Irion K, Felicetti JC, Camargo JJ, et al. Magnetic resonance imaging of pulmonary nodules: accuracy in a granulomatous disease-endemic region. 2015. Eur Radiol. 2016;26(9):2915-20. https://doi.org/10.1007/s00330-015-4125-1
22. Hochhegger B, de Souza VV, Marchiori E, Irion KL, Souza AS Jr, Elias Junior J, et al. Chest magnetic resonance imaging: a protocol suggestion. Radiol Bras. 2015;48(6):373-80. https://doi.org/10.1590/0100-3984.2014.0017