ABSTRACT
Objective: To determine whether pulmonary mechanics are altered in mice with sepsis. Methods: A total of 40 Balb/c mice were divided into two groups: survival (n = 21) and pulmonary mechanics (n = 19). The survival group was divided into three subgroups: control (n = 7), sublethal (n = 7) and lethal (n = 7). The pulmonary mechanics group was also divided into three subgroups: control (n = 5), sublethal (n = 7) and lethal (n = 7). Sepsis was induced through cecal ligation and puncture, the latter varying in degree (sublethal or lethal). At eight hours after the intervention, pulmonary mechanics were measured through end-inflation occlusion. In the pulmonary mechanics group, the following variables were studied: total pressure, resistance, viscoelasticity, dynamic compliance and static compliance. The data obtained were analyzed using one-way ANOVA. Results: The data for the survival group indicate the efficacy of the model employed. There were no statistically significant differences among the pulmonary mechanics subgroups in terms of dynamic compliance, static compliance, total pressure, resistance or viscoelasticity. Conclusion: At eight hours after cecal ligation and puncture, there were no changes in the lung parenchyma, nor were any alterations observed in the viscous and viscoelastic components of the lung.
Keywords:
Sepsis; Respiratory mechanics; Lung/injuries; Punctures/instrumentation; Respiratory distress syndrome, adult; Mice
RESUMO
Objetivo: Verificar se há alterações na mecânica pulmonar de camundongos com sepse. Métodos: Foram utilizados 40 camundongos Balb/c divididos em dois grupos: sobrevida (n = 21) e mecânica respiratória (n = 19). O grupo sobrevida foi dividido em três subgrupos: controle (n = 7), subletal (n = 7) e letal (n = 7). O grupo mecânica respiratória também foi dividido em três subgrupos: controle (n = 5), subletal (n = 7) e letal (n = 7). A sepse foi induzida pelo método cecal ligation and puncture, usando-se um estímulo subletal e outro letal. A mecânica pulmonar foi medida oito horas após a intervenção, utilizando-se o método da oclusão ao final da inspiração. Dentro do grupo mecânica pulmonar foram estudadas as seguintes variáveis: variação total de pressão, pressão resistiva, pressão viscoelástica, elastância dinâmica e elastância estática. Os dados foram analisados por meio do teste estatístico ANOVA One-Way. Resultados: Os dados do grupo sobrevida determinaram a eficácia do modelo utilizado. Não houve diferença estatística entre os subgrupos da mecânica pulmonar quando analisadas as elastâncias dinâmica e estática, bem como não houve diferença estatística entre os subgrupos da mecânica pulmonar quando analisadas a variação total de pressão, pressão resistiva e pressão viscoelástica. Conclusão: Não houve lesão estrutural no pulmão, bem como não houve alteração nos componentes viscoso e viscoelástico do pulmão quando essas variáveis foram estudadas oito horas após a intervenção pelo método cecal ligation and puncture.
Palavras-chave:
Sepse; Mecânica respiratória; Pulmão/lesões; Punções/instrumentação; Síndrome do desconforto respiratório do adulto; Camundongos
INTRODUCTIONSepsis-related pulmonary dysfunction is known to occur in humans as well as in animal models.(1-3) Sepsis is one of the leading causes of death in critically ill patients in the USA, where 750,000 individuals per year develop this syndrome, which leads to death in more than 210,000 of those individuals.(4-5) Sepsis from bacterial infection or endotoxemia is a primary cause of acute respiratory distress syndrome, since it induces intensive endogenous mediator activity, followed by leukocyte activation, intravascular inflammation, and cardiopulmonary failure.(3)
Two types of acute lung injury have been described: that of pulmonary origin and that of extrapulmonary origin.(6) In the present study, we will concentrate on acute respiratory distress syndrome of extrapulmonary origin, which initiates in an extrapulmonary focus, from where the inflammatory mediators depart in order to act upon the systemic circulation. In the lung, vascular endothelial cells are the first to suffer damage, resulting in increased vascular permeability, microcirculation congestion, interstitial edema, and a relative reduction in the size of the intra-alveolar spaces.(6-7)
Multiple organ failure is a common consequence in patients with the severe acute form of sepsis syndrome, and has been well described in the literature on the subject.(8-9) However, although much has been said about lung injury in sepsis, and a reasonable number of studies have been conducted, studies investigating the development of pulmonary edema present conflicting results on the development of pulmonary edema.(2-3,8-13) In addition, there have been few studies of the respiratory mechanics involved.(14)
The impact that alterations in respiratory mechanics caused by acute or chronic inflammation have on respiratory function has been well described in the literature.(15-17) It is known that pulmonary edema, although having various etiologies, can result from increased pulmonary vascular permeability, and that such edema causes changes in lung compliance, as well as in lung elasticity.(15-16) However, it remains unclear whether there are changes in respiratory mechanics when the model of choice is cecal ligation and puncture (CLP) in mice.
METHODSThe present study was an experimental study, and the study design was approved by the Ethics in Animal Research Committee of the University of Brasília.
A total of 40 male Balb/c wild-type mice, weighing 27 ± 2 g, were obtained from the animal facilities of the University of Brasília Veterinary Hospital. The animals were allocated to one of two groups: the survival group (n = 21) or the respiratory mechanics group (n = 19). The animals in the survival group were divided into three subgroups: sublethal CLP (SL-CLP, n = 7), lethal CLP (L-CLP, n = 7), and control (CTRL, n = 7). The animals in the respiratory mechanics group were also divided into three subgroups: SL-CLP (n = 7), L-CLP (n = 7), and CTRL (n = 5).
Sepsis was induced through CLP. The animals in both groups were anesthetized with a solution of ketamine (150 mg.kg-1, i.p.) and xylazine (7.5 mg.kg-1, i.p.). After the animals had been subjected to trichotomy of the abdominal region, they were placed on the surgical table for small animals. Asepsis of the abdominal region was performed using an iodine/alcohol solution. Subsequently, a 1-cm incision was made in the abdominal region, the cecum was exposed and then tied, near the ileocecal valve, with sterile silk suture, resulting in semi-occlusion of the intestinal flow. At that point, the cecum of each animal in the two SL-CLP subgroups was thrice perforated with a sterile needle (13 × 4.5), whereas those of the animals of the L-CLP subgroups were each perforated twelve times with a sterile needle (0.8 × 25), and those of the animals in the CTRL subgroups were not perforated. In the present study, we chose to include the SL-CLP and the L-CLP subgroups, since, despite the fact that the authors of studies employing this model of sepsis have made no such distinction,(10-11,22-23) the inflammatory stimulus in the SL-CLP subgroup allows the organic reaction and guarantees survival, in contrast to the inflammatory stimulus in the L-CLP subgroup, which, due to its magnitude, allows no organic reaction and makes survival impossible in this subgroup. After the above-mentioned procedures had been carried out, in the SL-CLP as well as in the L-CLP subgroups, the cecum of each mouse was gently squeezed in order to expel the fecal content. The cecum of each mouse was placed back into the peritoneal cavity, which was sutured using 4-0 silk and cleansed using 10% hydrogen peroxide. The animals were hydrated with a 1-mL subcutaneous dorsal injection of saline solution and were placed in a properly heated box for recovery.
The animals in the survival group were divided into three subgroups: SL-CLP, L-CLP, and CTRL. After the animals in these three subgroups had been submitted to the above-mentioned procedure, they were housed in the animal facilities, with free access to food and water, for 144 h. The animals were observed every 12 h in order to determine the number of animals that remained alive.
The animals in the respiratory mechanics group were divided into three subgroups: SL-CLP, L-CLP and CTRL. At 8 h after CLP, each animal was anesthetized and properly placed on the surgical table, where a small longitudinal incision was made in the anterior region of the neck. The adjacent tissues were spread until the trachea was exposed. At that point, a longitudinal incision was made between the two fibrous rings in order to introduce a tracheostomy tube (for small animals). Subsequently, the animal was taken to the registration point, where the tracheostomy tube was connected to a pneumotachograph.(19) Each animal then received a dose (3 µl per cavity, i.p.) of neuromuscular blocking agent (Pancuronium) and was mechanically ventilated with a respiratory frequency of 100 breaths per minute, a flow of 1.1 mL.s-1, a volume of 0.3 mL, and a positive end-expiratory pressure (PEEP) of 2 cmH2O. Once the pneumotachograph had been connected, the animal was on mechanical ventilation, and the neuromuscular junctions had been inhibited, the anterior chest wall was surgically removed. From that point on, it was possible to use end-inflation occlusion to measure the variables studied.
The pneumotachograph used had three lateral outlets. Two of those outlets were connected to a differential pressure transducer, which registered the airflow provided to the animal. Due to the low flow and the reduced dimensions of the trachea, the existing flow was laminar and could therefore be measured using Poiseuille's Law. The remaining lateral outlet was connected to an absolute pressure transducer, which registered the tracheal pressure. The volume was obtained through the electronic integration of the flow signal.
The mechanical ventilator was fed by an air compressor. Using this ventilator made it possible to control the inspiratory and expiratory times in order to adjust the respiratory frequency, as well as the end-inspiratory pause in order to carry out end-inflation occlusion. The volume was adjusted by altering the ratio between respiratory frequency and flow, the latter being controlled with a flowmeter.
The signals were obtained by the transducers that were connected to a signal conditioner and an analog-digital converter, being transferred, through the latter, to a computer. In the computer, the signals were registered using the WinDaq/Lite Data Acquisition software program, version 3.03.
Respiratory mechanics were measured through end-inflation occlusion.(20-21) This method was chosen due to the possibility of separating the respiratory system pressure into its viscous (rP1), viscoelastic (rP2), and elastic (Pel) components. Each animal was mechanically ventilated, and, at the end of each inhalation, the flow was occluded. After the occlusion, tracheal pressure decreases sharply until reaching a point of inflection, then decreasing gradually until reaching a plateau, which is equivalent to the lung elastic recoil pressure. Using these reference points for pressure, it was possible to calculate the variables studied in the present study.
We analyzed the following variables: total variation in pulmonary pressure, rPtot, L = rP1, L + rP2, L, considering rPtot, L (cmH2O) as the total variation in pulmonary pressure, rP1, L (cmH2O) as pulmonary resistance, and rP2, L (cmH2O) as lung viscoelasticity; pulmonary resistance, rP1, L = Ptot, L - Pi, L, considering rP1, L (cmH2O) as pulmonary resistance, Ptot, L (cmH2O) as total pulmonary pressure, and Pi, L (cmH2O) as pressure at the inflection point on the pulmonary pressure curve; lung viscoelasticity, rP2, L = Pi, L - Pel, L, considering rP2, L (cmH2O) as lung viscoelasticity, Pi, L (cmH2O) as pressure at the inflection point on the pulmonary pressure curve, and Pel, L (cmH2O) as lung elasticity; lung dynamic compliance, Cdyn, L = (Pi, L - PEEP) / V, considering Cdyn, L (cmH2O.mL-1) as lung dynamic compliance, Pi, L (cmH2O) as pressure at the inflection point of the pulmonary pressure curve, PEEP (cmH2O) as PEEP, and V (mL) as lung volume; and lung static compliance, Cstat, L = (Pel, L - PEEP) / V, considering Cstat, L (cmH2O.mL-1) as lung static compliance, Pel, L (cmH2O) as lung elasticity, PEEP (cmH2O) as PEEP, and V (mL) as lung volume.
All data are expressed as mean ± standard deviation. Analysis of variance was used to analyze the data obtained, and values of p < 0.05 were considered significant.
RESULTSTable 1 shows the data related to the variables 'flow', 'volume', and 'PEEP', as well as their distribution among the groups.
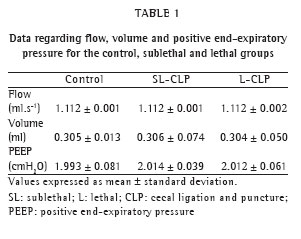
Figure 1 shows the survival curve for the three groups. After the intervention, all of the groups received the same treatment. Within the first 24 h after the intervention, the survival rate was 100% among the animals in the CTRL group, as well as among those in the SL-CLP group. However, all of the animals in the L-CLP group died during this period.
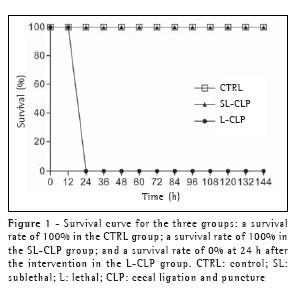
Regarding the total variation in pressure, we obtained 2.323 ± 0.108 cmH2O in the CTRL group, compared with 2.584 ± 0.410 cmH2O in the SL-CLP group and 2.446 ± 0.212 cmH2O in the L-CLP group.
Figure 2 displays a comparison of the variation in total pressure in the three groups. Regarding the viscous component of the lung, we obtained a pressure variation of 1.501 ± 0.067 cmH2O in the CRTL group, compared with 1.699 ± 0.340 cmH2O in the SL-CLP group and 1.614 ± 0.234 cmH2O in the L-CLP group. However, the pressure variation in the viscoelastic component was 0.822 ± 0.062 cmH2O in the CRTL group, compared with 0.885 ± 0.087 cmH2O in the SL-CLP group and 0.832 ± 0.063 cmH2O in the L-CLP group.
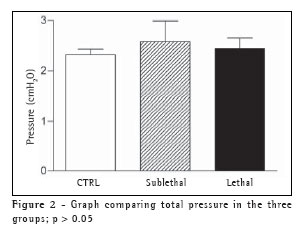
Figure 3 compares pulmonary resistance (energy dissipation in the viscous component of the lung) and lung viscoelasticity (energy dissipation in the viscoelastic component of the lung) in the CRTL, SL-CLP, and L-CLP groups. There were no statistically significant differences in the above-mentioned comparisons.

Dynamic compliance was 40.267 ± 3.141 cmH2O.mL-1 in the CRTL group, compared with 47.681 ± 20.999 cmH2O.mL-1 in the SL-CLP group and 40.833 ± 3.309 cmH2O.mL-1 in the L-CLP group. Static compliance was 38.919 ± 2.082 cmH2O.mL-1 in the CRTL group, compared with 45.622 ± 19.493 cmH2O.mL-1 in the SL-CLP group and 39.352 ± 2.757 cmH2O.mL-1 in the L-CLP group.
The comparison between dynamic and static compliance in the CTRL, SL-CLP, and L-CLP groups revealed no statistically significant differences (Figure 4).

DISCUSSIONWe found no differences between the groups in any of the variables studied. In the literature, we found some studies that drew a correlation between the CLP-induced sepsis model and pulmonary edema.(10-11,22-23) In that correlation, there is evidence in terms of the null hypothesis as well as of the alternative hypothesis. Therefore, our results regarding the null hypothesis are in agreement with those reported in some studies in the literature(10,22-23) if we consider pulmonary edema to imply subsequent alteration in respiratory mechanics.(24-25)
The comparison of the ?Ptot, L (corresponding to the total variation in pulmonary pressure) and the rP1, L (corresponding to the energy dissipation in the viscous component of the lung) revealed no differences among the three groups, which is in agreement with other published studies.(10,22,23) This demonstrates that the airways are patent and that, if any change in pulmonary structure had been observed in the present study, this alteration would probably have occurred in the tissues. The rP2, L corresponds to the energy dissipation in the viscoelastic component of the lung, and this is related to two phenomena: pendelluft and stress relaxation.(26) The viscoelastic component is related to the pulmonary structure, which was probably unaffected in the present study and did not differ among the CTRL, SL-CLP, and L-CLP groups.
In one study(14) data regarding respiratory mechanics in models of sepsis were presented. However, the method used to induce sepsis in that study (Escherichia coli endotoxin) was different from that used in our study. The authors of that study demonstrated that there is a decrease in pulmonary compliance at 12 h after the intervention but observed no changes in airway resistance. Those results are in disagreement with the findings of the present study, in which no differences were observed among the CTRL, SL-CLP, and L-CLP groups in terms of Cdyn, L and Cstat, L at 8 h after the induction of sepsis. In the present study, no alterations were observed in Cdyn, L or Cstat, L. We attribute this fact to the rapidity with which the animals died, since, as has been widely described in the literature, sepsis leads to a pronounced, uncontrolled increase in the production of chemical mediators, which in turn leads to multiple organ failure.(13)
It is known that intervention can provide an inflammatory stimulus of such magnitude that the microbicidal activity of the inflammatory cells that migrated to the focus of the lesion is suppressed by the increased production of nitric oxide.(27-29) This increased nitric oxide production is beneficial at first. However, after the activation of inducible nitric oxide synthase has increased it to the point of overproduction, it begins to inhibit the microbicidal activity of the inflammatory cells, especially that of neutrophils.(27-29) Therefore, we are led to understand that there is not sufficient time for lesion of the pulmonary structure and the consequent attempt at repair to occur, since the chemical change is so great that death precedes any possible structural lesion.
We conclude that there are no changes in the lung parenchyma, nor any alterations in the viscous or viscoelastic components of the lung, at 8 h after CLP-related induction of sepsis. We suggest that another model of sepsis induction be used in order to study sepsis-related pulmonary alterations.
REFERENCES 1. Kristof AS, Goldberg P, Laubach V, Hussain SN. Role of inducible nitric oxide synthase in endotoxin-induced acute lung injury. Am J Respir Crit Care Med. 1998; 158(6):1883-9.
2. McCaffree DR, Gray BA, Pennock BE, Coalson J, Bridges C, Taylor FB, et al. Role of pulmonary edema in the acute pulmonary response to sepsis. J Appl Physiol. 1981;50(6):1198-205.
3. Ayala A, Chung CS, Lomas JL, Song GY, Doughty LA, Gregory SH, et al. Shock-induced neutrophil mediated priming for acute lung injury in mice: divergent effects of TLR-4 and TLR-4/FasL deficiency. Am J Pathol. 2002;161(6):2283-94.
4. Angus DC, Linde-Zwirble WT, Lidicker J, Clermont G, Carcillo J, Pinsky MR. Epidemiology of severe sepsis in the United States: analysis of incidence, outcome, and associated costs of care. Crit Care Med. 2001;29(7):1303-10. Comment in: Crit Care Med. 2001;29(7):1472-4.
5. Murphy SL. Deaths: Final data for 1998. Natl Vital Stat Rep. 2000;24(11):1-105.
6. Pelosi P, Gattinoni L. Acute respiratory distress syndrome of pulmonary and extra-pulmonary origin: fancy or reality? Intensive Care Med. 2001;27(3):457-60. Comment on: Intensive Care Med. 2001;27(3):477-85.
7. Rocco PR, Zin WA. Pulmonary and extrapulmonary acute respiratory distress syndrome: are they different? Curr Opin Crit Care. 2005;11(1):10-7.
8. Cohen J. The immunopathogenesis of sepsis. Nature 2002;420(6917):885-91.
9. Hotchkiss RS, Karl IE. The pathophysiology and treatment of sepsis. N Engl J Med. 2003;348(2):138-50. Comment in: N Engl J Med. 2003;348(16):1600-2; author reply 1600-2.
10. Rackow EC, Astiz ME, Janz TG, Weil MW. Absence of pulmonary edema during peritonitis and shock in rats. J Lab Clin Med. 1988;112(2):264-9.
11. Stephens KE, Ishizaka A, Larrick JW, Raffin TA. Tumor necrosis factor causes increased pulmonary permeability and edema. Comparison to septic acute lung injury. Am Rev Respir Dis. 1988;137(6):1364-70.
12. Martin GS, Bernard GR; International Sepsis Forum. Airway and lung in sepsis. Intensive Care Med. 2001;27 Suppl 1:S63-79.
13. Callery MP, Kamei T, Mangino MJ, Flye MW. Organ interactions in sepsis. Host defense and the hepatic-pulmonary macrophage axis. Arch Surg. 1991;126(1):28-32.
14. Castiello A, Paterson JF, Shelley SA, Haller EM, Balis JU. Depletion of surfactant tubular myelin with pulmonary dysfunction in a rat model for acute endotoxemia. Shock. 1994;2(6):427-32.
15. Peták F, Habre W, Hantos Z, Sly PD, Morel DR. Effects of pulmonary vascular pressures and flow on airway and parenchymal mechanics in isolated rat lungs. J Appl Physiol. 2002;92(1):169-78.
16. Parker JC, Gillespie MN, Taylor AE, Martin SL. Capillary filtration coefficient, vascular resistance, and compliance in isolated mouse lungs. J Appl Physiol. 1999;87(4):1421-7.
17. Macedo-Neto AV, Santos LV, Menezes SL, Paiva DS, Rocco PR, Zin WA. Respiratory mechanics after prosthetic reconstruction of the chest wall in normal rats. Chest. 1998;113(6):1667-72.
18. Baker CC, Chaudry IH, Gaines HO, Baue AE. Evaluation of factors affecting mortality rate after sepsis in a murine cecal ligation and puncture model. Surgery. 1983;94(2):331-5.
19. Mortola JP, Noworaj A. Two-sidearm tracheal cannula for respiratory airflow measurements in small animals. J Appl Physiol 1983;55(1 Pt 1):250-3.
20. Bates JHT, Rossi A, Milic-Emili J. Analysis of the behavior of the respiratory system with constant inspiratory flow. J Appl Physiol. 1985;58(6):1840-8.
21. Bates JHT, Baconnier P, Milic-Emili J. A theoretical analysis of interrupter technique for measuring respiratory mechanics. J Appl Physiol. 1988;64(5):2204-14.
22. Ferrari-Baliviera E, Mealy K, Smith RJ, Wilmore DW. Tumor necrosis factor induces adult respiratory distress syndrome in rats. Arch Surg. 1989;124(12):1400-5.
23. Piper RD, Pitt-Hyde M, Li F, Sibbald WJ, Potter RF. Microcirculatory changes in rat skeletal muscle in sepsis. Am J Respir Crit Care Med. 1996;154(4 Pt 1):931-7.
24. Barnas GM, Watson RJ, Green MD, Sequeira AJ, Gilbert TB, Kent J, et al. Lung and chest wall mechanical properties before and after cardiac surgery with cardiopulmonary bypass. J Appl Physiol. 1994;76(1):166-75.
25. Barnas GM, Stamenovic D, Lutchen KR. Lung and chest wall impedances in the dog in normal range of breathing: effects of pulmonary edema. J Appl Physiol. 1992;73(3):1040-6.
26. Horie T, Hildebrandt J. Dynamic compliance, limit cycles, and static equilibria of excised cat lung. J Appl Physiol. 1971;31(3):423-30.
27. Crosara-Alberto DP, Darini ALC, Inoue RY, Silva JS, Ferreira SH, Cunha FQ. Involvement of NO in the failure of neutrophil migration in sepsis induced by Staphylococcus aureus. Br J Pharmacol. 2002;136(5):645-58.
28. Benjamim CF, Silva JS, Fortes ZB, Oliveira MA, Ferreira SH, Cunha FQ. Inhibition of leukocyte rolling by nitric oxide during sepsis leads to reduced migration of active microbicidal neutrophils. Infect Immun. 2002;70(7):3602-10.
29. Benjamim CF, Ferreira SH, Cunha FQ. Role of nitric oxide in the failure of neutrophil migration in sepsis. J Infect Dis. 2000;182(1):214-23.
_________________________________________________________________________________________
* Study carried out at the Universidade de Brasília (UnB, University of Brasilia), Brasília, Distrito Federal, Brazil.
1. Graduate student at the Universidade de Brasília (UnB, University of Brasilia), Brasília, Distrito Federal, Brazil
2. Physiotherapy degree from the Universidade de Brasília (UnB, University of Brasilia), Brasília, Distrito Federal, Brazil
3. Masters from the Universidade de Brasília (UnB, University of Brasilia), Brasília, Distrito Federal, Brazil
4. Physiotherapist; Doctoral student at the Universidade de Brasília (UnB, University of Brasilia), Brasília, Distrito Federal, Brazil
5. Pulmonology degree from the Universidade de Brasília (UnB, University of Brasilia), Brasília, Distrito Federal, Brazil
6. Professor at the Universidade de Brasília (UnB, University of Brasilia), Brasília, Distrito Federal, Brazil
Correspondence to: Rodrigo Storck Carvalho. CLSW 303, Bloco A, Apto 154, Setor Sudoeste, Cruzeiro - CEP 70673-621, DF, Brasil. Tel 55 61 3202-0347. E-mail rodrigo.storck@terra.com.br
Submitted: 18 July 2005. Accepted, after review: 17 October 2005.