ABSTRACT
Objective: To characterize the presence, extent, and patterns of dynamic hyperinflation (DH) during treadmill exercise testing in patients with moderate to severe COPD. Methods: This was a cross-sectional study involving 30 non-hypoxemic patients (FEV1= 43 ± 14% of predicted) who were submitted to a cardiopulmonary exercise test on a treadmill at a constant speed (70-80% of maximum speed) to the tolerance limit (Tlim). Serial inspiratory capacity (IC) maneuvers were used in order to assess DH. Results: Of the 30 patients studied, 19 (63.3%) presented with DH (DH+ group), having greater pulmonary function impairment at rest than did those without DH (DH− group). None of the variables studied correlated with exercise tolerance in the DH− group, whereas Tlim, IC, and perception of dyspnea during exercise did so correlate in the DH+ group (p < 0.05). In the DH+ group, 7 and 12 patients, respectively, presented with a progressive and a stable pattern of DH (ΔICTlim,2min = −0.28 ± 0.11 L vs. 0.04 ± 0.10 L; p < 0.01). Patients with a progressive pattern of DH presented with higher perception of dyspnea/Tlim rate and lower exercise tolerance than did those with a stable pattern (354 ± 118 s and 465 ± 178 s, respectively; p < 0.05). Conclusions: The presence of DH is not a universal phenomenon during walking in COPD patients, even in those with moderate to severe airflow limitation. In the patients who presented DH, a progressive pattern of DH had a greater impact on exercise tolerance than did a stable pattern of DH.
Keywords:
Pulmonary disease, chronic obstructive; Exercise; Exercise test; Inspiratory capacity.
RESUMO
Objetivo: Caracterizar a presença, extensão e padrões de hiperinsuflação dinâmica (HD) durante teste em esteira rolante em pacientes com DPOC moderada a grave. Métodos: Estudo transversal com 30 pacientes não hipoxêmicos (VEF1= 43 ± 14% do previsto) submetidos a teste cardiopulmonar de exercício em esteira rolante em velocidade constante (70-80% da velocidade máxima) até o limite da tolerância (Tlim). Manobras seriadas de capacidade inspiratória (CI) foram utilizadas para avaliação da HD. Resultados: Dos 30 pacientes estudados, 19 (63,3%) apresentaram HD (grupo HD+), que apresentaram maior comprometimento funcional em repouso do que os pacientes sem HD (grupo HD−). Nenhuma das variáveis obtidas relacionou-se com a tolerância ao exercício no grupo HD−, enquanto Tlim, CI e percepção de dispneia ao esforço foram significativamente correlacionados no grupo HD+ (p < 0,05). No grupo HD+, 7 e 12 pacientes, respectivamente, apresentaram padrão progressivo e estável de HD (ΔCITlim,2min = −0,28 ± 0,11 L e 0,04 ± 0,10 L; p < 0,01). Pacientes com padrão progressivo de HD apresentaram maior relação percepção de dispneia/Tlim e menor tolerância ao exercício do que aqueles com padrão estável (354 ± 118 s e 465 ± 178 s, respectivamente; p < 0,05). Conclusões: A HD não é um fenômeno universal durante a caminhada em pacientes com DPOC, mesmo que apresentem obstrução ao fluxo aéreo de graus moderado a acentuado. Nos pacientes que apresentaram HD, um padrão progressivo de HD teve maior repercussão na tolerância ao exercício do que um padrão estável de HD.
Palavras-chave:
Doença pulmonar obstrutiva crônica; Exercício; Teste de esforço; Capacidade inspiratória.
IntroductionExercise intolerance, notably dynamic exercise intolerance, plays a fundamental role in COPD-associated morbidity, as well as being related to increased health care costs and mortality.(1) Although the reduction in exercise capacity in COPD has a multifactorial origin,(2-4) the progressive increase in operating lung volumes, secondary to expiratory flow limitation (EFL)-dynamic hyperinflation (DH)-and the consequent dyspnea, takes on a major role, especially in patients with moderate to severe disease.(2)
From a clinical standpoint, the simplest and most effective strategy to evaluate DH during cardiopulmonary exercise testing is to monitor inspiratory capacity (IC), assuming that as long as TLC does not vary considerably,(5) IC will be inversely related to end-expiratory lung volume (EELV).(6-8) However, nearly all of the studies evaluating DH during exercise in patients with COPD paradoxically employed a cycle ergometer, having generally evaluated DH in response to constant workload protocols.(6-10) Nevertheless, ventilatory demand and exercise-induced hypoxemia are known to be greater during walking, and there are distinct patterns of recruitment of accessory respiratory muscles.(11-13) In contrast, cycle ergometer exercise tends to trigger greater leg fatigue, ventilatory rates that are sufficiently high for the DH process to be truly limiting being therefore more difficult to achieve.(14-16) In addition, there is an extensive body of knowledge demonstrating that the physiological adjustments in the two exercise modalities do not overlap; in fact, the responses to interventions aimed at improving respiratory mechanics seem to be more clearly observed during walking.(15) Another complicating factor is that recent studies have demonstrated that the kinetic behavior of DH is more complex than it was previously thought to be, some patients either avoiding(17) or delaying(18) DH development during cycle ergometer exercise. However, all of the abovementioned aspects remain largely unexplored during ambulation in patients with COPD.
The principal objective of the present study was to determine the presence, extent, and possible development of distinct patterns of DH during a walk test in patients with moderate to severe COPD. In order to mimic constant workload cycle ergometer tests and allow the extrapolation of the findings to clinical exercise tests, we chose to evaluate IC during tests on a treadmill at constant speed.
MethodsThirty male patients over 40 years of age with COPD, as determined by the Global Initiative for Chronic Obstructive Lung Disease criteria,(1) presenting with post-bronchodilator FEV1 < 70% of predicted and reporting a history of smoking (> 20 pack-years) were invited to participate in the study. The patients were recruited from among those being treated at the COPD outpatient clinic or the pulmonary rehabilitation center of the institution. We included patients who did not present with any of the following:
comorbidities that might lead to dyspnea and exercise limitation
COPD exacerbation or respiratory infection in the previous month
need for continuous oxygen therapy or an SaO2 < 90% at rest
contraindication for clinical exercise testing
The study project was approved by the local research ethics committee.
After an initial visit, during which the clinical and functional characteristics of the patients were determined by spirometry (performed prior to and after the administration of 400 µg of inhaled albuterol), the patients returned for two more experimental visits. During one of such visits, an incremental cardiopulmonary test was performed, and, during the other (occurring at least 72 h after the previous visit), a constant speed test was performed at 70-80% of the maximum speed achieved during the incremental cardiopulmonary test. In order to avoid the acute effects of the bronchodilators on the ventilatory responses to exercise, the patients performed the constant speed tests without having previously received bronchodilators. Continuous long-acting bronchodilators were discontinued 12 h prior to the exercise tests (in the case of 2-adrenergic agonists) or 24 h prior to the tests (in the case of anticholinergics).
The spirometric tests were performed with a Koko PFT spirometer (PDS Instrumentation, Inc., Louisville, CO, USA). The variables measured included FVC, FEV1, FEV1/FVC ratio, and IC. Maximal voluntary ventilation was estimated by multiplying FEV1 by 37.5.(19) The patients completed at least three slow, forced expiratory maneuvers, considered acceptable and reproducible. The predicted values were derived from those for the Brazilian population.(20)
The cardiopulmonary exercise tests were performed with the patients connected to a Vmax 229cT system (SensorMedics, Yorba Linda, CA, USA) via a face mask and walking on an ATL treadmill (Inbrasport, Porto Alegre, RS, Brazil). During the incremental test, after 2 min at a constant speed of 1.6 km/h without inclination, the speed was increased every 1 min by 0.3 km/h, 0.5 km/h, or 0.8 km/h depending on the functional capacity of the patient, as determined by the examiner prior to the test. During the tests, the patients were instructed to hold the side bars only when needed (dizziness and loss of balance, among others). During the constant speed test, after a 2-min warm-up phase (similar to that of the incremental test), the work rate was suddenly increased to a speed corresponding to 70-80% of the maximum speed achieved during the incremental test, and the patients were encouraged to walk until they reached their tolerance limit, the time to reach it (Tlim) being measured. At the end of the initial phase and every 2 min during the tests, the patients were asked about the intensity of dyspnea and leg discomfort, by means of the modified Borg scale.(21)
The following variables were measured (breath by breath) and expressed as mean 15-s time in absolute values and percentage of predicted(22):
oxygen consumption (VO2), in mL/min, under standard temperature, pressure dry (STPD) conditions
carbon dioxide release, in mL/min, under STPD conditions
minute ventilation under body temperature, pressure saturated conditions
tidal volume
RR
The gas exchange threshold was evaluated by the V-slope method.(23)
The R-R interval on a 12-lead electrocardiogram was used in order to determine HR, and pulse oximetry with an Onyx 9500T pulse oximeter (Nonin, Plymouth, MN, USA) was used in order to estimate SpO2. We evaluated the dynamic changes in operating lung volumes by serial IC measurements, assuming that TLC remained constant during exercise.(5) The validity and reproducibility of that procedure, as well as its sensitivity to interventions, have been extensively demonstrated in patients with COPD.(6-10) Two maneuvers were performed at rest, at the end of the initial period and every 2 min after the beginning of the constant speed test, in order to obtain reproducible values (< 10% difference in relation to the highest value, at each stage). We defined DH as any reduction in IC in relation to the initial values at rest.(24)
The data are presented as means and standard deviations for the variables with normal distribution and as medians and ranges for those with non-normal distribution. The Statistical Package for the Social Sciences, version 13.0 (SPSS Inc., Chicago, IL, USA), was used. Possible differences between groups were investigated by unpaired t-test or the Mann-Whitney test, when appropriate. Pearson's test was used in order to evaluate the correlations among continuous variables, and the chi-square test was used in order to evaluate the correlations among categorical variables. Two-way repeated measures ANOVA was used in order to compare the different groups of patients in terms of IC throughout the tests. The level of statistical significance was set at 5% for all tests (p < 0.05).
ResultsAs shown in Table 1, the patients generally presented with moderate to severe obstructive lung disease and reduced FVC. Of the 30 patients, 24 (80%) presented with IC reductions that are typically related to EFL at rest (< 80% of predicted).(25) As expected, all of the patients presented with airflow limitation on exertion, dyspnea being the principal symptom limiting exercise. The gas exchange threshold was identified in 10 patients and corresponded to 51.3 ± 10.1% of the maximal O2 predicted, values that were within or slightly below the expected range for the age bracket and gender.
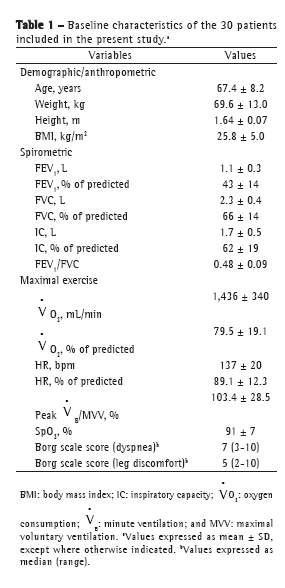
Of the 30 patients, 19 (63.3%) presented with a significant reduction in IC and were therefore included in the DH+ group, whereas 11 presented with no reduction in IC or even with an increase in IC and were therefore included in the DH− group (Table 2). The patients in the DH+ group tended to present with greater pulmonary function impairment at rest. However, pre-exercise IC was greater in the DH+ group, having abruptly decreased in the rest-exercise transition (Table 2). The DH+ and DH- groups were markedly similar in terms of maximal exercise capacity (Table 2). In contrast, submaximal exercise capacity (Tlim) was significantly lower in the DH+ group, and the dyspnea scores corrected for the duration of the exercise were higher (Table 2). Statistically significant correlations among the variations in IC, Tlim, and dyspnea score at the end of the test were found only in the patients in the DH+ group (Figure 1). In the patients in whom we identified the gas exchange threshold, we found that the tests were generally performed at an intensity that was equivalent to that parameter (p > 0.05), those patients being equally distributed into the two groups (5 patients in each group).
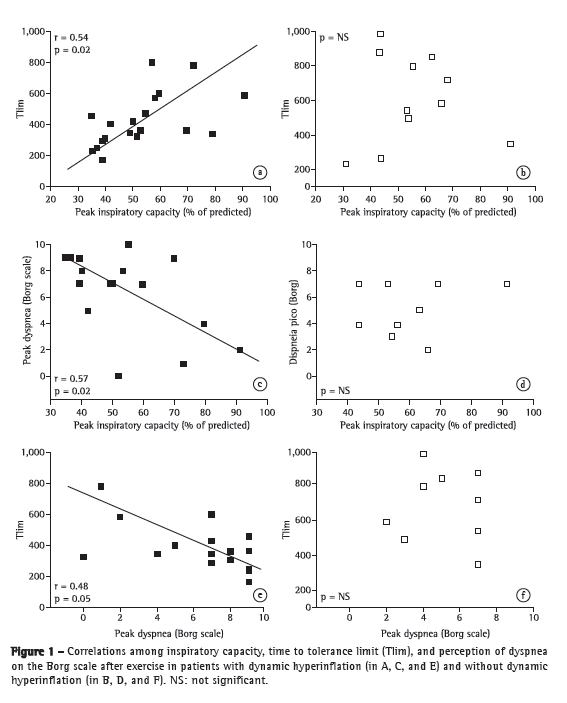
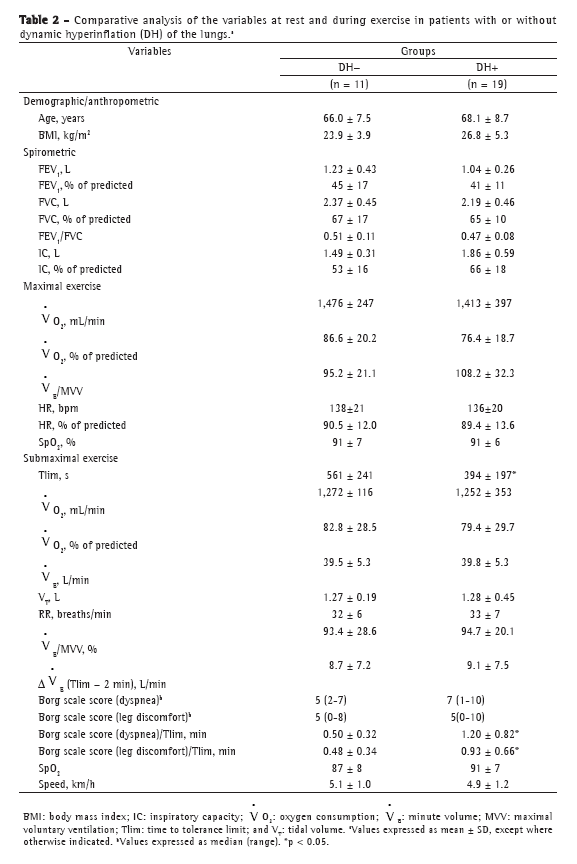
A more detailed analysis of the behavior of operating lung volumes in the DH+ group revealed a pronounced difference in the pattern of reduction in IC throughout the test. In the DH+ group, although IC decreased in the rest-exercise transition in all of the patients, beginning at 2 min after the initiation of the test, 7 patients presented with a progressive reduction in IC, whereas the remaining patients presented with stable IC, meaning that we identified patients with a progressive and a stable pattern of DH (ICTlim,2min = −0.28 ± 0.11 L vs. 0.04 ± 0.10 L; p < 0.01; Figure 2). As shown in Table 3, the patients with a progressive pattern of DH tended to present with greater pulmonary function impairment at rest. However, there were no differences between the patients with progressive DH and those with stable DH except for a lower Tlim value and higher symptom scores corrected for the duration of the exercise in the former (p < 0.05). There were no differences between the groups in terms of the magnitude of variation in the ventilatory response throughout the test (Table 3) or the respiratory pattern (p > 0.05; data not shown).
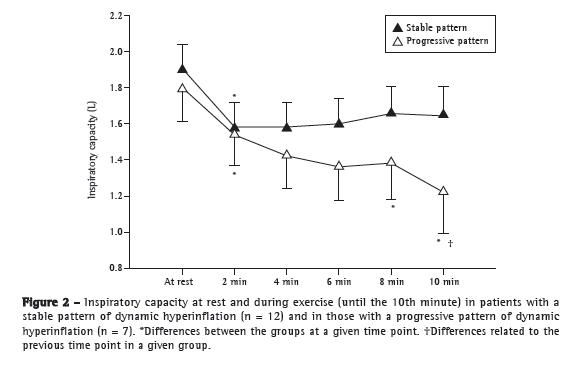
DiscussionThe present study investigated the behavior of operating lung volumes, as estimated by variations in IC, during treadmill walking in patients with moderate to severe COPD. The original results can be summarized as follows:
In one third of the sample, DH was not demonstrated, which indicates that DH is not a universal phenomenon in COPD patients, even in those in whom there is clear evidence of airflow obstruction at rest.
Spirometric changes were inadequate predictors of the presence and extent of DH on exertion, although DH tended to correlate with greater pulmonary function impairment at rest.
Even in the patients who presented with DH, the kinetic pattern of such changes was heterogeneous, with patterns of progressive and stable hyperinflation.
A pattern of progressive hyperinflation determined lower exercise tolerance and, possibly, greater perception of dyspnea than did a pattern of stable hyperinflation, as suggested by comparing the groups in terms of the perception of dyspnea corrected for the duration of the exercise performed (Borg scale/Tlim).
These data provide new evidence that can aid in understanding the responses of respiratory mechanics during walking in patients with COPD and that allow us to reflect on the mechanisms underlying the heterogeneity of the responses to pharmacological and nonpharmacological interventions that characterizes this population,(1,6-10) although the methods employed in the present study do not allow us to draw definitive conclusions regarding this issue.
The fundamental pathophysiological determinants of exercise intolerance in COPD are traditionally related to a chronic imbalance between an increased ventilatory demand-due to an increase in the dead space/tidal volume ratio, increased neural drive, and hypoxemia, among others-and a reduced capacity to meet that demand.(26) In addition, most of the patients with COPD, especially those with moderate to severe airflow obstruction, present with DH, accompanied by progressive air trapping and increased EELV. This phenomenon is particularly expected in patients with EFL (i.e., patients who present with increased transpulmonary pressure without a proportional increase in airflow). In those patients, increased ventilatory requirements during exercise almost invariably condition some degree of DH.(2,6-8) Therefore, DH allows patients, when ventilating at larger volumes in a given inspiratory duty cycle (inspiratory time/total respiratory time ratio), to increase their ability to generate expiratory flows in order to maintain an alveolar ventilation that is closer to that which is needed in order to meet an increased metabolic demand.
Although the exact nature of the control of such adjustments remains controversial,(2,4) it is likely that the basic mechanism is time-dependent, with increased airflow resistance (i.e., increased mean pulmonary time constant) and loss of lung compliance, which determines an expiratory time that is progressively insufficient for the exhalation of the volume inhaled previously. As a consequence, volume balance is not achieved, and functional residual capacity is dictated dynamically, positive end-expiratory pressures being generated. Therefore, tidal volume transforms into progressively higher lung volumes that are typically located in the least compliant portion of the pressure-volume curve. One of the consequences of such changes is that there is a significant increase in the work of breathing and a reduction in the ability of the diaphragm to generate effective pressures, leading to a progressive neuromechanical dissociation and dyspnea.(2,6-10) Dyspnea can also be induced by EFL per se, probably through reflexes originating from the dynamic compression of the intrathoracic airways.(27)
The findings of the present study corroborate the concept that DH plays an important role as an etiological factor for exercise limitation in patients with moderate to severe COPD. Therefore, two thirds of the patients evaluated in the present study were unequivocally DH+ (Table 2), and the extent of that phenomenon correlated with exercise intolerance and the consequent dyspnea (Figure 1). It is of note that, despite the lack of statistical significance, the patients in the DH+ group tended to present with disease that was more severe than was that seen in those in the DH− group, which is in agreement with the notion that a more limited flow reserve at rest translates to a greater tendency to develop DH.(28,29) A surprising finding was a greater pre-exercise IC in the DH+ group (Table 2); however, this should be interpreted with extreme caution, given that, as will be discussed below, the absence of static lung volume measurements does not allow us to rule out the possibility that EELV was actually higher in those patients, meaning that TLC could be substantially higher in the patients in the DH+ group than in those in the DH- group. In contrast, the hypothesis that that finding represented nothing more than a "regression to the mean" phenomenon-IC having decreased only in those patients with high IC at rest-is inconsistent with the data shown in Table 2 and Figure 1, meaning that the reduction in IC effectively had biological significance in that group.
In comparison with supported exercise (as is the case of cycle ergometer exercise), walking results in an earlier and more abrupt increase in ventilatory overload. Therefore, it is reasonable to assume that the elastic and resistive work during exercise is particularly prominent in that type of ergometric exercise.(12) In this context, increased minute ventilation associated with a higher RR while walking could result in shorter expiratory time, leading to greater dynamic air trapping in comparison with that observed during cycle ergometer exercise. In addition, the movement of the arms during dynamic exercise performed in the upright position causes the intercostal inspiratory muscles to contribute less to respiratory mechanics than they do during cycle ergometer exercise because they are actively participating in the support of the arms and trunk(30); the abdominal muscles also aid in stabilizing the trunk, being less efficient in expiratory lung emptying(31); walking is associated with a less efficient gas exchange and a worsening of ventilation/perfusion mismatch, probably as a result of postural differences among lung volumes, respiratory mechanics, or lower mixed venous oxygen tension(12); the mechanoreceptors located in the upper limbs provide more afferent stimulation to the respiratory center(12); and larger muscle groups are recruited.(13)
Although the present study did not directly compare walk tests and cycle ergometer exercise in terms of their ability to induce DH, a surprising finding was that one third of the patients under study showed no reduction in IC during exercise. Initially, it would be reasonable to assume that a more stable ventilatory response or respiratory pattern in that group led to the maintenance of operating lung volumes during exercise. However, that did not occur, and there were no differences in hypoxic drive (Table 3). Therefore, the most probable explanation is that EFL either became less extensive or did not develop during exercise in the DH- group.(28,29) Alternatively, it is possible that abdominal muscle recruitment was increased in those patients, thereby increasing expiratory flow rates,(17,18) given that there were no significant differences between the groups regarding ventilatory demand and respiratory patterns (Table 2). In fact, abdominal muscle fatigue seems to be a common phenomenon in patients in whom the severity of COPD is similar to that observed in the patients investigated in the present study.(32) Unfortunately, because neither the expiratory time nor the inspiratory time during exercise was available, we were unable to evaluate the ventilatory flows. Further studies employing techniques that directly measure expiratory muscle activity(32) or estimate it, such as optoelectronic plethysmography,(33) can provide additional evidence in order to explain those findings.
From a clinical standpoint, it should be highlighted that serial IC measurements have been widely used in order to investigate DH on exertion and DH response to interventions in patients with COPD.(6-10) In this context, our findings indicating that some patients do not present with DH (Table 2) whereas others present with a stable pattern of DH (Figure 2) provide a mechanistic basis for the observation of the heterogeneity of exercise response in COPD patients. Although that aspect was not formally investigated in the present study, we hypothesize that patients without DH benefit less from interventions that reduce the DH rate than do those with a stable pattern of DH, and that the latter benefit less from such interventions than do those with the highest potential for improvement, i.e., those with a progressive pattern of DH. Further studies are needed in order to confirm that. In the present study, we found that the perception of leg fatigue was greater among the patients with a progressive pattern of DH than among those with a stable pattern of DH, although the difference was not statistically significant (Table 3). There is evidence suggesting that oxygen delivery to the appendicular muscles during exercise is reduced in patients with COPD,(34) and some of the interventions that reduce respiratory muscle overload, such as the use of bronchodilators(35) and noninvasive positive pressure ventilation,(36) are associated with a reduced sensation of leg discomfort. Therefore, we speculate that because of greater respiratory muscle overload, oxygen delivery to the legs of patients with progressive DH is impaired, which causes greater sensation of discomfort.
The principal limitation of the present study was the fact that we did not measure static lung volumes at rest. This means that the variations in lung volumes were estimated exclusively by IC, not by EELV (i.e., TLC/IC). Although this limitation did not allow us to evaluate, in an adequate manner, possible differences in the baseline degree of positioning of operating lung volumes, isolated measurements of IC are commonly used in clinical practice and have high external validity. However, we recognize that this introduces a major element of uncertainty regarding the y axis intercept of operating lung volumes at rest, which means that caution is recommended when interpreting the IC values at rest as indicators of hyperinflation. In addition, our data should not be extrapolated to hypoxemic patients, in whom the hypoxic drive can modulate the kinetics of DH development. We also recognize that our study might not have enough statistical power to demonstrate the differences between the subgroups. This seems to indicate that future studies addressing such aspects should involve a substantially higher number of patients. Finally, the walk test on a treadmill at constant speed does not faithfully mimic walking as an activity of daily living; however, we should emphasize that, in general, the speed developed (approximately 5 km/h) represents a possible compromise, i.e., a test that achieves an intensity that is not too low (which would make it difficult to interpret Tlim) while maintaining some degree of external validity.
In conclusion, the results of the present study indicate that DH is not a universal phenomenon during treadmill walking in COPD patients, even in those who present with moderate to severe airflow obstruction. In the patients who present with DH, a pattern of progressive reduction in operating lung volumes has a greater impact on exercise tolerance than does a stable pattern of DH. Additional studies are needed in order to measure the impact of such a heterogeneous response to pharmacological and nonpharmacological interventions in patients with COPD.
References1. Global Initiative for Chronic Obstructive Lung Disease; National Heart,
Lung, and Blood Institute. Global strategy for the diagnosis, management, and prevention of chronic obstructive pulmonary disease. Bethesda: National Institutes of Health, National Heart, Lung, and Blood Institute; 2006.
2. O'Donnell DE, Webb KA. The major limitation to exercise performance in COPD is dynamic hyperinflation. J Appl Physiol. 2008;105(2):753-5; discussion 755-7.
3. Debigaré R, Maltais F. The major limitation to exercise performance in COPD is lower limb muscle dysfunction. J Appl Physiol. 2008;105(2):751-3; discussion 755-7.
4. Aliverti A, Macklem PT. The major limitation to exercise performance in COPD is inadequate energy supply to the respiratory and locomotor muscles. J Appl Physiol. 2008;105(2):749-51; discussion 755-7.
5. Stubbing DG, Pengelly LD, Morse JL, Jones NL. Pulmonary mechanics during exercise in subjects with chronic airflow obstruction. J Appl Physiol. 1980;49(3):511-5.
6. O'Donnell DE, Webb KA. Exertional breathlessness in patients with chronic airflow limitation. The role of lung hyperinflation. Am Rev Respir Dis. 1993;148(5):1351-7.
7. O'Donnell DE, Lam M, Webb KA. Measurement of symptoms, lung hyperinflation, and endurance during exercise in chronic obstructive pulmonary disease. Am J Respir Crit Care Med. 1998;158(5 Pt 1):1557-65.
8. O'Donnell DE, Revill SM, Webb KA. Dynamic hyperinflation and exercise intolerance in chronic obstructive pulmonary disease. Am J Respir Crit Care Med. 2001;164(5):770-7.
9. Neder JA, Fuld JP, Overend T, Thirlwell J, Carter R, Stevenson R, et al. Effects of formoterol on exercise tolerance in severely disabled patients with COPD. Respir Med. 2007;101(10):2056-64.
10. Berton DC, Reis M, Siqueira AC, Barroco AC, Takara LS, Bravo DM, et al. Effects of tiotropium and formoterol on dynamic hyperinflation and exercise endurance in COPD. Respir Med. 2010;104(9):1288-96.
11. Weiler-Ravell D, Cooper DM, Whipp BJ, Wasserman K. Control of breathing at the start of exercise as influenced by posture. J Appl Physiol. 1983;55(5):1460-6.
12. Palange P, Forte S, Onorati P, Manfredi F, Serra P, Carlone S. Ventilatory and metabolic adaptations to walking and cycling in patients with COPD. J Appl Physiol. 2000;88(5):1715-20.
13. Miyamura M, Honda Y. Oxygen intake and cardiac output during treadmill and bicycle exercise. J Appl Physiol. 1972;32(2):185-8.
14. Man WD, Soliman MG, Gearing J, Radford SG, Rafferty GF, Gray BJ, et al. Symptoms and quadriceps fatigability after walking and cycling in chronic obstructive pulmonary disease. Am J Respir Crit Care Med. 2003;168(5):562-7.
15. Pepin V, Saey D, Whittom F, LeBlanc P, Maltais F. Walking versus cycling: sensitivity to bronchodilation in chronic obstructive pulmonary disease. Am J Respir Crit Care Med. 2005;172(12):1517-22.
16. Saey D, Debigare R, LeBlanc P, Mador MJ, Cote CH, Jobin J, et al. Contractile leg fatigue after cycle exercise: a factor limiting exercise in patients with chronic obstructive pulmonary disease. Am J Respir Crit Care Med. 2003;168(4):425-30.
17. Aliverti A, Stevenson N, Dellacà RL, Lo Mauro A, Pedotti A, Calverley PM. Regional chest wall volumes during exercise in chronic obstructive pulmonary disease. Thorax. 2004;59(3):210-6.
18. Vogiatzis I, Georgiadou O, Golemati S, Aliverti A, Kosmas E, Kastanakis E, et al. Patterns of dynamic hyperinflation during exercise and recovery in patients with severe chronic obstructive pulmonary disease. Thorax. 2005;60(9):723-9.
19. Neder JA, Andreoni S, Lerario MC, Nery LE. Reference values for lung function tests. II. Maximal respiratory pressures and voluntary ventilation. Braz J Med Biol Res. 1999;32(6):719-27.
20. Pereira CA, Sato T, Rodrigues SC. New reference values for forced spirometry in white adults in Brazil. J Bras Pneumol. 2007;33(4):397-406.
21. Borg GA. Psychophysical bases of perceived exertion. Med Sci Sports Exerc. 1982;14(5):377-81.
22. Neder JA, Nery LE, Castelo A, Andreoni S, Lerario MC, Sachs A, et al. Prediction of metabolic and cardiopulmonary responses to maximum cycle ergometry: a randomised study. Eur Respir J. 1999;14(6):1304-13.
23. Beaver WL, Wasserman K, Whipp BJ. A new method for detecting anaerobic threshold by gas exchange. J Appl Physiol. 1986;60(6):2020-7.
24. Johnson BD, Weisman IM, Zeballos RJ, Beck KC. Emerging concepts in the evaluation of ventilatory limitation during exercise: the exercise tidal flow-volume loop. Chest. 1999;116(2):488-503.
25. Diaz O, Villafranca C, Ghezzo H, Borzone G, Leiva A, Milic-Emil J, et al. Role of inspiratory capacity on exercise tolerance in COPD patients with and without tidal expiratory flow limitation at rest. Eur Respir J. 2000;16(2):269-75.
26. Nery LE, Wasserman K, French W, Oren A, Davis JA. Contrasting cardiovascular and respiratory responses to exercise in mitral valve and chronic obstructive pulmonary diseases. Chest. 1983;83(3):446-53.
27. O'Donnell DE, Sanii R, Anthonisen NR, Younes M. Effect of dynamic airway compression on breathing pattern and respiratory sensation in severe chronic obstructive pulmonary disease. Am Rev Respir Dis. 1987;135(4):912-8.
28. Dellacà RL, Duffy N, Pompilio PP, Aliverti A, Koulouris NG, Pedotti A, et al. Expiratory flow limitation detected by forced oscillation and negative expiratory pressure. Eur Respir J. 2007;29(2):363-74.
29. Koulouris NG, Dimopoulou I, Valta P, Finkelstein R, Cosio MG, Milic-Emili J. Detection of expiratory flow limitation during exercise in COPD patients. J Appl Physiol. 1997;82(3):723-31.
30. Couser JI Jr, Martinez FJ, Celli BR. Respiratory response and ventilatory muscle recruitment during arm elevation in normal subjects. Chest. 1992;101(2):336-40.
31. Heijdra YF, Dekhuijzen PN, van Herwaarden CL, Folgering HT. Effects of body position, hyperinflation, and blood gas tensions on maximal respiratory pressures in patients with chronic obstructive pulmonary disease. Thorax. 1994;49(5):453-8.
32. Hopkinson NS, Dayer MJ, Moxham J, Polkey MI. Abdominal muscle fatigue following exercise in chronic obstructive pulmonary disease. Respir Res. 2010;11:15.
33. Cala SJ, Kenyon CM, Ferrigno G, Carnevali P, Aliverti A, Pedotti A, et al. Chest wall and lung volume estimation by optical reflectance motion analysis. J Appl Physiol. 1996;81(6):2680-9.
34. Aliverti A, Macklem PT. How and why exercise is impaired in COPD. Respiration. 2001;68(3):229-39.
35. Berton DC, Barbosa PB, Takara LS, Chiappa GR, Siqueira AC, Bravo DM, et al. Bronchodilators accelerate the dynamics of muscle O2 delivery and utilisation during exercise in COPD. Thorax. 2010;65(7):588-93.
36. Borghi-Silva A, Oliveira CC, Carrascosa C, Maia J, Berton DC, Queiroga F Jr, et al. Respiratory muscle unloading improves leg muscle oxygenation during exercise in patients with COPD. Thorax. 2008;63(10):910-5.
Study carried out at the ABC School of Medicine, Santo André, Brazil.
Correspondence to: Elie Fiss. Avenida Príncipe de Gales, 821, CEP 09060-650, Santo André, SP, Brasil.
Tel/Fax: 55 11 4438-3558. E-mail: eliefiss@uol.com.br
Financial support: None.
Submitted: 5 October 2011. Accepted, after review: 5 December 2011.
About the authorsPriscila Kessar Cordoni
Assistant Professor. Department of Pulmonology, ABC School of Medicine, Santo André, Brazil.
Danilo Cortozi Berton
Adjunct Professor. Institute of Health Sciences, Feevale University, Novo Hamburgo, Brazil.
Selma Denis Squassoni
Physical Therapist in Charge. Department of Pulmonology, ABC School of Medicine, Santo André, Brazil.
Maria Enedina Aquino Scuarcialupi
Professor. Paraíba School of Medicine and Medical Sciences, João Pessoa, Brazil.
José Alberto Neder
Adjunct Professor. Department of Pulmonology, ABC School of Medicine, Santo André, Brazil; and Full Professor. Pulmonology Section, Department of Medicine, Federal University of São Paulo, São Paulo, Brazil.
Elie Fiss
Full Professor. Department of Pulmonology, ABC School of Medicine, Santo André, Brazil.