ABSTRACT
Sleep is essential for the proper functioning of all individuals. Sleep-disordered breathing can occur at any age and is a common reason for medical visits. The objective of this consensus is to update knowledge about the main causes of sleep-disordered breathing in adult and pediatric populations, with an emphasis on obstructive sleep apnea. Obstructive sleep apnea is an extremely prevalent but often underdiagnosed disease. It is often accompanied by comorbidities, notably cardiovascular, metabolic, and neurocognitive disorders, which have a significant impact on quality of life and mortality rates. Therefore, to create this consensus, the Sleep-Disordered Breathing Department of the Brazilian Thoracic Association brought together 14 experts with recognized, proven experience in sleep-disordered breathing.
Keywords:
Sleep apnea syndromes/diagnosis; Sleep apnea syndromes/therapy; Hypoventilation.
RESUMO
O sono é essencial para o adequado funcionamento de todos os indivíduos. Os distúrbios respiratórios do sono ocorrem em todas as faixas etárias, constituindo motivo frequente de consulta médica. O objetivo deste consenso foi atualizar os conhecimentos sobre os principais distúrbios respiratórios do sono tanto na população adulta quanto na pediátrica, com ênfase na apneia obstrutiva do sono. A apneia obstrutiva do sono é uma doença extremamente prevalente, porém frequentemente subdiagnosticada. Associa-se frequentemente a uma série de comorbidades, notadamente cardiovasculares, metabólicas e neurocognitivas, que impactam significativamente na qualidade de vida e na mortalidade. Por conta disso, o Departamento de Distúrbios Respiratórios do Sono da Sociedade Brasileira de Pneumologia e Tisiologia reuniu 14 especialistas com reconhecida e comprovada experiência em distúrbios respiratórios do sono para a elaboração deste documento.
Palavras-chave:
Síndromes da apneia do sono/diagnóstico; Síndromes da apneia do sono/terapia; Hipoventilação.
INTRODUCTION Sleep is essential for the health and well-being of children, adolescents, and adults, being important for cognitive function, as well as for mental, cardiovascular, cerebrovascular, and metabolic health.(1) Sleep can be affected by various respiratory disorders. Among the causes of sleep-disordered breathing (SDB), the most prevalent is obstructive sleep apnea (OSA), which is characterized by frequent upper airway (UA) collapse during sleep, resulting in intermittent hypoxia and sleep fragmentation.(2) In most cases, OSA has cardiovascular, metabolic, and neurocognitive consequences, with a significant decrease in quality of life,(2,3) as well as increasing the risk of death. (4) When left untreated, OSA constitutes a health risk, with economic costs that affect the individuals with the condition, their families, and society.(5,6)
OBSTRUCTIVE SLEEP APNEA Prevalence Data from the Wisconsin Sleep Cohort Study, which involved workers between 30 and 60 years of age, demonstrated that the prevalence of OSA in men and women was 24% and 9%, respectively, when the criterion was an apnea-hypopnea index (AHI) ≥ 5 events/h, compared with 9% and 4%, respectively, when the criterion was an AHI ≥ 15 events/h.(7) However, in recent years, there has been a clear increase in the prevalence of OSA,(8-10) possibly due to the aging of the population, higher rates of obesity, the development of methods of detection that are more sensitive, such as the use of nasal-cannula-pressure-transducer systems (instead of the exclusive use of thermistors), and more “tolerant” hypopnea criteria (desaturation of 3% rather than 4%). In a study conducted in the city of São Paulo, Brazil, involving 1,042 volunteers representative of the adult population, 32.8% of the participants were diagnosed with OSA syndrome, which is characterized by an AHI ≥ 5 events/h with symptoms or an AHI ≥ 15 events/h irrespective of symptoms.(11) Another study found that the prevalence of an AHI ≥ 5 events/h ranged from 9% to 38% in the general population, reaching rates as high as 90% and 78% among men and women, respectively, in certain elderly populations.(12) In one study comparing White and Asian subjects matched for age, sex, and BMI, the severity of OSA was found to be greater in the latter, probably due to differences in craniofacial anatomy.(13)
Recent estimates suggest that, worldwide, nearly one billion people have OSA, moderate/severe OSA affecting an estimated 425 million individuals between 30 and 69 years of age.(14) Brazil is among the ten countries with the highest estimated number of individuals with OSA(14): 49 million with an AHI ≥ 5 events/h; and 25 million with an AHI ≥ 15 events/h. In certain population groups, OSA is highly prevalent, such groups including individuals in the preoperative period of bariatric surgery,(15,16) those with resistant hypertension,(17,18) those with atrial fibrillation,(19) and those who have had a stroke.(20)
Insomnia and OSA are highly prevalent sleep disorders. The combination of the two diseases, which is also common and is known as comorbid insomnia and sleep apnea, impairs quality of life and reduces adherence to positive airway pressure treatment, as well as increasing mortality rates.(21-23)
Pathophysiology In humans, the UAs are responsible for speech, swallowing, and ventilation; because they are composed of numerous muscles and soft parts that do not have a protective bone framework, they are predisposed to collapse.(24) Although that ability is essential for speech and swallowing when an individual is awake, it also allows the UAs to collapse during sleep, resulting in OSA.(24) The pathophysiology of OSA is complex and may involve four main phenotypes (Chart 1).(25)
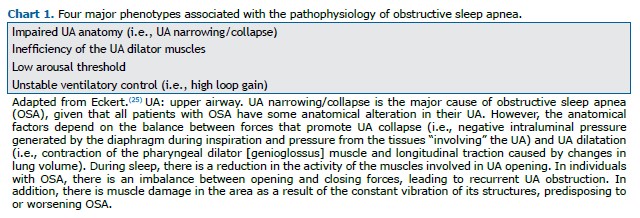
Another factor that plays a role in the genesis of OSA is unstable ventilatory control, which can lead to cyclical respiratory effort and periodic breathing. This abnormal respiratory effort can produce variable levels of negative intraluminal pressure and an inadequate compensatory response from the dilator muscles, predisposing to UA collapse. Brief arousals followed by apnea cause increased respiratory effort and changes in ventilation, resulting in fluctuations in PaO2 and PaCO2 levels and instability of respiratory control centers, perpetuating a cyclical breathing pattern.(26)
Episodes of obstructive apnea can lead to intermittent hypoxia and mild CO2 retention, disrupting normal autonomic and hemodynamic responses during sleep.(27) Occurring repeatedly during the night and accompanied by a chemoreceptor-mediated increase in sympathetic activity, episodes of obstructive apnea increase the sympathetic activity of peripheral blood vessels, with consequent vasoconstriction.(27) That hemodynamic stress occurs when there is severe hypoxemia and hypercapnia, and it may initiate pathophysiological mechanisms that promote various diseases, via sympathetic activation, increased release of vasoactive substances, systemic inflammation, and oxidative stress.(27)
Obesity is the main cause of UA narrowing and is therefore a major predictor of OSA.(25) The pathophysiology of OSA in obesity is a multifactorial process, in which the main mechanism may be the deposition of adipose tissue in the structures of the neck, leading to luminal narrowing and UA collapse. (15,16) In addition, obese individuals with OSA often have more tongue fat than do similarly obese individuals without OSA.(28) Increasing body weight accelerates the progression of OSA, whereas weight loss reduces in its severity.(29) Obesity can also reduce lung volume, promoting UA collapse,(30) and is often associated with greater neck circumference (NC). A larger NC is associated with an increased risk of OSA.(31,32) The NC, which is typically larger in men than in women, correlates with waist circumference, BMI, metabolic syndrome, and cardiovascular risk factors.(32-34) The nocturnal displacement of fluid from the legs to the neck may contribute to UA collapse, and that displacement has been shown to correlate significantly with the AHI, NC, and time spent sitting when awake.(35)
It is known that OSA is more common in men than in women, and that its prevalence is higher in postmenopausal women than in those who are premenopausal.(36,37) In comparison with women, men have a longer pharyngeal airway and a greater cross-sectional area of the soft palate, indicating that the UA is more collapsible in men than in women.(38) Other potential contributing factors include the deleterious effects of male sex hormones and the protective effects of female sex hormones.(39) Progesterone stimulates the UA muscles and ventilation and may contribute to the lower prevalence of OSA in premenopausal women,(40) whereas higher testosterone levels (due to androgen supplementation or polycystic ovary disease) can worsen OSA.(41,42)
The prevalence of OSA increases with age,(10,43) possibly due to the following mechanisms(44,45): increased fat deposition around the pharynx; loss of tissue elasticity; lengthening of the soft palate; and decreased respiratory chemoreceptor responses. In elderly individuals, OSA may go undiagnosed because of the common misperception that the symptoms are due to aging rather than to OSA. The prevalence of OSA tends to be similar among aging men and women, especially among those over 60 years of age.(10,43)
Clinical consequences In individuals with OSA, there can cardiovascular, metabolic, and neurocognitive consequences, as well as other, less common, repercussions (Chart 2).(2,46-50) Especially in its more severe forms, OSA is associated with several cardiovascular comorbidities, such as stroke,(20,51) coronary artery disease,(52) hypertension,(18) and arrhythmias (notably atrial fibrillation).(19) Regular treatment with CPAP can reduce cardiovascular risk.(53-55)
The increased production of oxidants and inflammatory mediators, caused by obstructive events, together with the increase in left ventricular afterload, also contribute to altering cardiac electrophysiology, with remodeling of the heart chambers and a consequent increase in the risk of arrhythmias, especially atrial fibrillation. Bradyarrhythmias, ventricular arrhythmias, and atrioventricular conduction abnormalities may also occur, especially when OSA and hypoxemia are more severe.(56,57)
The prevalence of hypertension in individuals with OSA is significant (as high as 50%), and approximately 30% of patients with hypertension have OSA.(50) In individuals with resistant hypertension, the prevalence of OSA has been reported to be as high as 80%.(17) Constant activation of the sympathetic nervous system is the likely pathway responsible for the increase in blood pressure; intermittent hypoxia, negative intrathoracic pressure, and primary hyperaldosteronism may also be involved.(58,59) Many individuals with OSA do not show the expected nocturnal drop in blood pressure, being classified as having OSA with a “nondipping” pattern (a drop in blood pressure of less than 10% during sleep).(57,60)
Another aspect of OSA is its association with stroke. The reported prevalence of OSA in individuals who have a stroke ranges from 30% to 70%.(61) The chance of having a stroke has been shown to be higher among individuals with an AHI ≥ 20 events/h than among those with an AHI < 5 events/h, even after adjustment for confounders (OR = 4.33; 95% CI: 1.32-14.24).(62) The cascade of deleterious pathophysiological events that occurs in OSA probably contributes to the concomitant occurrence of arrhythmias, oxidative stress, endothelial dysfunction, atherosclerosis, hypertension, autonomic dysfunction, and hypercoagulability. In individuals who have had a stroke, OSA leads to worse clinical outcomes(61,63): longer hospital stays and prolonged rehabilitation; increased risk of stroke recurrence, and a greater risk of death.
Metabolic syndrome is strongly associated with OSA, with a consequent increase in cardiovascular risk.(64) The clinical features of metabolic syndrome—central obesity, hypertension, insulin resistance, hyperglycemia, and dyslipidemia—are shared by patients with OSA.(65) A study encompassing two cohorts found an increased incidence of metabolic syndrome associated with OSA,(66) and treatment of OSA with CPAP may reduce some components of metabolic syndrome, such as blood pressure and triglyceride levels.(67) In a recent randomized study of patients with OSA and metabolic syndrome, the proportion of patients in whom metabolic syndrome was reversed was greater among those who were treated with CPAP than among those who were not, although that reversal occurred in only a minority of the patients, suggesting that there is a need for combined treatments.(68) Despite robust evidence of the interplay between the two conditions, OSA is still underdiagnosed in individuals with the metabolic syndrome.(64,69) A diagnosis of OSA is a risk factor for the development of type 2 diabetes mellitus, and OSA leads to poorer glycemic control in individuals who develop the disease.(70)
Residual excessive sleepiness, even after adequate treatment with CPAP, occurs in 12% to 30% of patients with OSA.(71) Other causes of excessive daytime sleepiness (EDS) should always be investigated, such as insufficient sleep, inadequate sleep hygiene, and restless legs syndrome, as well as clinical conditions such as hypothyroidism, depression, and narcolepsy. (72) When CPAP is the treatment for OSA, optimization is essential, because nonadherence to CPAP treatment is a major cause of residual sleepiness. In addition, OSA can result in a neurocognitive decline (mainly in executive functions, attention, and memory).(73) Although symptoms of depression are prevalent in patients with OSA, such depression may be associated with comorbidities such as obesity and metabolic syndrome.(74)
Another important complication is the increase in the frequency of automobile accidents in which individuals with OSA are at fault, mainly attributable to EDS. In the case of professional drivers, the risk of an automobile accident is even higher than in the general population, because they are more likely to have comorbidities, such as obesity, cardiovascular disease, diabetes mellitus, and metabolic syndrome. (75) The chance of causing an automobile accident is two to three times higher in individuals with untreated OSA than in individuals without OSA.(76)
In recent studies of the combination of OSA and cancer, the severity of OSA and intermittent nocturnal hypoxemia was found to be associated with increased tumor growth and aggressiveness, notably in cases of melanoma.(77,78)
Association with chronic lung diseases In many cases, OSA occurs in conjunction with chronic lung diseases, including interstitial lung disease, bronchial asthma (BA), COPD, and pulmonary hypertension. A systematic review and meta-analysis involving a collective total of 569 patients with interstitial lung disease showed that the prevalence of OSA in that population was 61%.(79) In another study, the prevalence of moderate-to-severe OSA was found to be higher in patients with idiopathic pulmonary fibrosis and a diagnosis of severe OSA was found to be strongly associated with the presence of cardiovascular disease, especially ischemic heart disease.(80) The combination of OSA and idiopathic pulmonary fibrosis worsens the prognosis, increases cardiovascular risk, and increases the risk of death; however, when individuals are regularly treated with CPAP (> 4 h/night), there is a significant improvement in EDS and sleep quality, as well as a reduction in the mortality rate.(80-82)
The prevalence of OSA can be high in patients with BA, ranging from 19% to 60% and being as high as 95% in those with severe BA.(83) In BA, inflammatory infiltration of the UAs and the increase in fat deposition on the walls of the pharynx, due to the use of corticosteroids or to obesity, lead to a reduced transverse UA diameter, thus favoring the occurrence of OSA.(83) In one study, the rate of decline in FEV1 was shown to be higher in patients with BA and OSA than in those with BA alone, the AHI being found to be the only independent risk factor for a decline in lung function.(84) However, among the patients with severe OSA, the rate of decline in FEV1 was found to be significantly lower in those who were treated with CPAP than in those who were not.(84) Another study evaluated the impact of prolonged CPAP treatment on clinical symptoms in individuals with BA and OSA: there was a significant reduction in BA symptoms and a reduction in the use of rescue medication.(85) A multicenter prospective study evaluating a collective total of 99 patients with BA and OSA demonstrated that, after 6 months of CPAP use, there were significant improvements in BA control, quality of life, and pulmonary function.(86) A systematic review of patients with BA and OSA treated with CPAP also documented improved quality of life, especially in those with severe OSA or uncontrolled BA.(87)
Prevalence studies of overlap syndrome (COPD in conjunction with OSA) tend to report a wide variation in results, mainly due to differences in definitions and in the populations studied, the reported prevalence of the syndrome ranging from 10% to 65%.(88) The different clinical phenotypes of COPD influence the chance of having OSA(89): increased lung volume and low BMI are associated with the emphysema phenotype and protect against OSA, whereas peripheral edema and high BMI, often associated with the chronic bronchitis phenotype, increase the risk of OSA.(89) Narrowing of the UAs, due to a fluid shift from the lower limbs (edema) to the neck (especially in those with cor pulmonale), and UA myopathy, due to COPD itself or the use of corticosteroids, are both factors that contribute to OSA.(89)
Classic OSA symptoms (e.g., snoring, morning headache, and EDS) and traditional risk factors for OSA (e.g., male gender, advanced age, and increased NC) are not helpful in confirming the suspicion of OSA in patients with COPD, particularly in those with moderate or severe COPD.(90,91) Therefore, in patients with COPD, polysomnography (PSG) is indicated when there is clinical suspicion of OSA, hypoxemic complications (cor pulmonale and polycythemia), or pulmonary hypertension disproportionate to the degree of airflow impairment.(92) Because OSA and COPD are clinical conditions associated with hypoxia and systemic inflammation, they increase cardiovascular risk and worsen other comorbidities, such as pulmonary hypertension; it is therefore to be expected that morbidity and mortality rates will be higher in patients with overlap syndrome than in those with COPD or OSA alone.(90,93) In an observational study of patients with overlap syndrome, the risk of COPD exacerbation and the mortality rate were both found to be lower among those who were treated with CPAP,(94) which is therefore indicated for the treatment of overlap syndrome. Similarly, CPAP treatment has been associated with increased survival in patients with moderate-to-severe OSA and COPD with hypoxemia who were on long-term supplemental oxygen therapy.(95)
Although there are limited data, it seems likely that SDB is more prevalent in adults with pulmonary hypertension than in the general adult population.(96-98) In individuals with OSA alone, there is a small increase in pulmonary artery pressure, usually without clinical significance.(99) In contrast, OSA in combination with obesity hypoventilation syndrome or COPD contributes to the development of significant pulmonary hypertension, which can be severe.(99) The population of patients with pulmonary hypertension typically differs from the general population with suspected OSA in that it has a predominance of women and a smaller proportion of individuals who are obese, those with nocturnal hypoxemia also having lower survival rates.(100) When indicated, CPAP treatment has the potential to improve pulmonary hemodynamics, although the decrease achieved in pulmonary artery pressure is minimal.(96)
Clinical suspicion The main risk factors for OSA are male gender, obesity, advanced age, and craniofacial abnormalities. (2,101,102) Anamnesis should be performed in order to investigate snoring/observed apnea (mainly in men) and tiredness/fatigue/morning headache/insomnia (mainly in women), as well as EDS.(101,103) One of the main daytime symptoms of OSA is EDS, which is often accompanied by cognitive and functional changes (difficulty concentrating, irritability, and impaired memory and work ability), as well as being associated with a higher rate of automobile accidents,(2,101) as detailed in Chart 3.
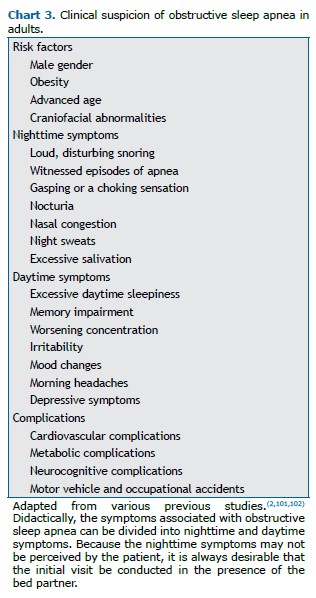
As part of the physical examination, clinicians should routinely evaluate blood pressure (to identify hypertension), BMI, NC, and craniofacial abnormalities (micrognathia, retrognathia, alterations of the soft palate, lateral narrowing of the oropharynx, hypertrophy of tonsils, and macroglossia), as well as the Mallampati score and nasal obstruction.(2,101,102) Systematic assessment of the pharynx using Friedman staging, including the modified Mallampati score and tonsil size,(104) is generally recommended as a predictor of success in soft tissue surgical treatment,(105,106) as shown in Chart 4.
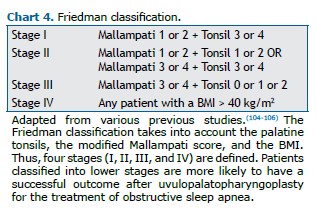
The various screening instruments for OSA are based on clinical, demographic, and anthropometric data, with the aim of identifying adult individuals at high risk for the disorder. It should be noted that no single instrument is capable of ruling in or ruling out OSA without an objective sleep study. The sensitivity and specificity of a screening instrument are inversely related.(107) In the case of OSA, a highly prevalent and often underdiagnosed disorder, it is perhaps more important that a screening instrument has high sensitivity and does not fail to diagnose patients with OSA, rather than having high specificity.(107) Due to the lack of a clear benefit in treating asymptomatic individuals, screening for OSA is not recommended in such individuals.(108)
One possible use of OSA screening instruments is to identify patients classified as being at high risk for OSA so that they can be referred for portable or home diagnostic methods, thus trimming the waiting lists at sleep laboratories.(109-111) Another possible use is in individuals in the preoperative period, given that patients with OSA are at increased risk of respiratory and cardiovascular complications in the postoperative period.(112,113) Comparing the performance of screening instruments can be particularly difficult because it depends on the type of objective sleep study used for diagnosis, the type of population studied, and the AHI cutoff used for diagnosis.(114,115)
Chief among the various screening instruments are the Berlin questionnaire,(116) the STOP-Bang questionnaire,(117) the NoSAS score,(118) and the GOAL questionnaire,(119) all of which are detailed in Chart 5.
The Epworth Sleepiness Scale (ESS), developed several decades ago,(120) is widely used in clinical practice. However, it is generally of low utility as a screening model for OSA, possibly because patients with OSA may not necessarily have EDS and because EDS may have many causes other than OSA,(121,122) as well as because the ESS is not reliably reproducible when applied sequentially.(123) The ESS has been validated for use in Brazil.(124)
Another OSA screening tool is the Berlin questionnaire,(116) which has also been validated for use in Brazil.(125) In a systematic review and meta-analysis, this instrument was found to have moderate sensitivity and low specificity for OSA in individuals evaluated at sleep clinics,(107) potentially performing better in a primary care setting than in sleep laboratories.(126)
The STOP-Bang questionnaire(117) has been widely validated: in a meta-analysis of studies involving individuals referred to sleep laboratories,(127) the questionnaire presented a sensitivity of 90%, 94%, and 96%, respectively, for the detection of any OSA, moderate/severe OSA, and severe OSA, whereas its specificity for those same parameters was 49%, 34%, and 25%, respectively. The STOP-Bang questionnaire has also been validated for use in Brazil.(128)
The NoSAS score was derived from a cohort in Switzerland and externally validated in a cohort in Brazil. (118) In both cohorts, NoSAS performed significantly better than did the STOP-Bang and Berlin questionnaires.(118) The NoSAS has been validated in various clinical settings, having proved to be a useful screening tool in all of those settings.(129)
The GOAL questionnaire, which uses only four dichotomous clinical parameters, was originally developed and validated for use in Brazil.(119) In the original study,(119) the GOAL questionnaire showed satisfactory performance in screening for OSA, with a discriminatory capacity similar to that obtained with the three other instruments evaluated (the No-Apnea score, STOP-Bang questionnaire, and NoSAS score). It was subsequently validated in other clinical contexts, always showing satisfactory performance.(130,131)
Laboratory diagnosis The various types of objective sleep studies are described in Chart 6. A type 1 sleep study (attended PSG) is considered the gold standard for the diagnosis of OSA and stratification of its severity.(57,132) However, it must be performed in a sleep laboratory by trained technical personnel, therefore being inherently costly, and is not widely available.(2,133) There can be night-to-night variability in the AHI data obtained by PSG, which may be related to the time spent in the supine position (in which the AHI is typically greater than in the lateral position) or to the use of alcohol and drugs that act on the central nervous system.(134,135)
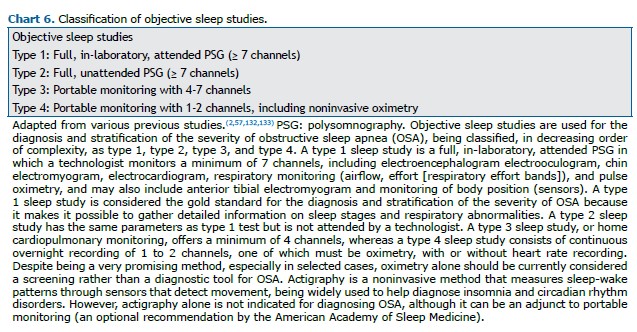
The combination of electroencephalography, electrooculography, and electromyography allows the staging of rapid eye movement (REM) sleep and non-REM sleep (subdivided into stages N1, N2, and N3),(133) as outlined in Chart 7. Respiratory parameters such as episodes of apnea, hypopnea, and respiratory effort-related arousals are measured with airflow sensors (a nasal-cannula-pressure-transducer system or an oronasal thermistor), through the quantification of respiratory effort (with chest straps and abdomen), and by determination of the degree of oxygenation (pulse oximetry). For certain diagnoses, it may be necessary to employ other techniques in order to measure some optional parameters, such as video monitoring, the use of an esophageal balloon to accurately determine the respiratory effort, and the measurement of expired CO2 (by capnography) or transcutaneous CO2 in cases of suspected hypoventilation.(57,132,133)
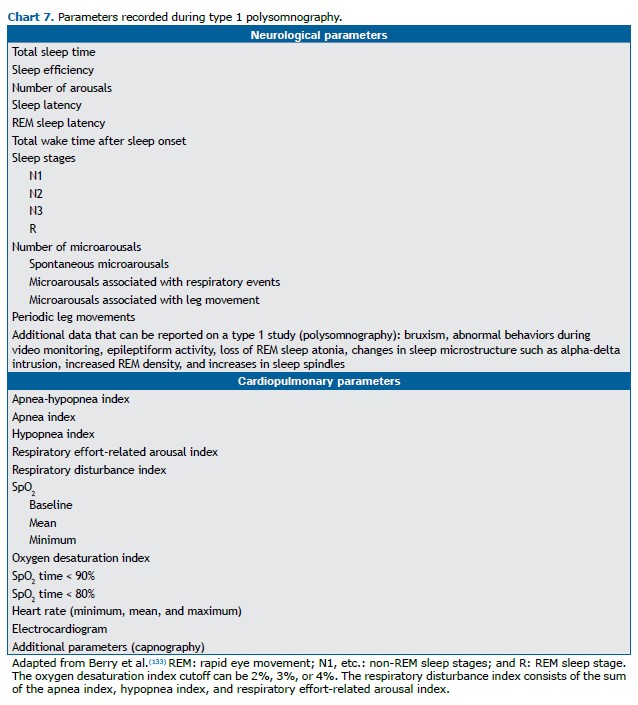
The results of a PSG are analyzed by a professional certified in the area, who stages sleep and evaluates respiratory events in accordance with the rules established by the American Academy of Sleep Medicine (AASM),(133) as detailed in Chart 8.
The currently recommended definition of hypopnea is at least a 30% reduction in flow, accompanied by 3% desaturation or a microarousal. However, there is an alternative definition that allows 4% desaturation without a microarousal. Therefore, variability of the hypopnea index in the same patient may result from the use of different criteria, and it is essential to describe which criteria were used to define hypopnea in the PSG report.(136) Differences in the definition of hypopnea can affect the AHI, and a lack of consistency in the definition of these events makes it difficult to interpret the results of a sleep study.(136,137)
Based on the degree of evidence established by the Grading of Recommendations Assessment, Development, and Evaluation (GRADE) method, the AASM has made a number of recommendations. 1) A PSG or home monitoring using portable polygraphy can be used for diagnosis in uncomplicated patients who have signs and symptoms that are indicative of moderate or high risk of OSA (strong recommendation). 2) A PSG should be performed when home testing is negative, inconclusive, or technically inappropriate (strong recommendation). 3) In the presence of significant cardiopulmonary disease, neuromuscular disease, waking hypoventilation (or suspected sleep hypoventilation), chronic opioid use, severe insomnia, or a history of stroke, a PSG should be performed (strong recommendation—preferred over home testing). It should be noted that, in relation to the use of home methods in the diagnosis of SDB in patients with neuromuscular diseases, there are studies that suggest that even nocturnal oximetry would be sufficient to assess these patients, especially when PSG is not feasible.(138,139) 4) A second PSG should be performed if the first is negative and a strong suspicion of OSA remains (weak recommendation). 5) Questionnaires alone should not be used for the diagnosis of OSA, an objective sleep test (PSG or polygraphy; strong recommendation) always being recommended.(2)
Split-night PSG is a diagnostic method in which, in the same examination, a baseline PSG is initially performed, followed by CPAP titration in the second half of the night. The AASM suggests that, if clinically appropriate, a split-night study can be used instead of a full-night PSG for the diagnosis of OSA (weak recommendation).(2) For a split-night study to be acceptable, CPAP titration should be initiated only when moderate or severe OSA is detected during at least 2 h of the diagnostic recording time, followed by at least 3 h of CPAP titration.(2)
Home diagnosis As described in Chart 9, portable OSA diagnostic devices were developed to reduce cost, increase patient comfort, and simplify the diagnostic process. As can be seen in Figure 1, type 3 handheld devices are recommended for the diagnosis of OSA in individuals with a high pretest probability for moderate-to-severe OSA who do not have severe or decompensated comorbidities(2,140) and should be managed at facilities with experience in sleep medicine, under the supervision of physicians certified in the specialty.(2)
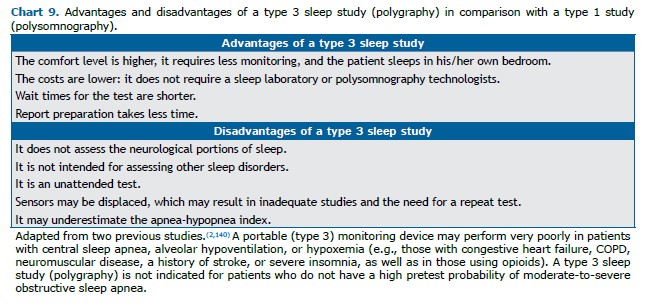
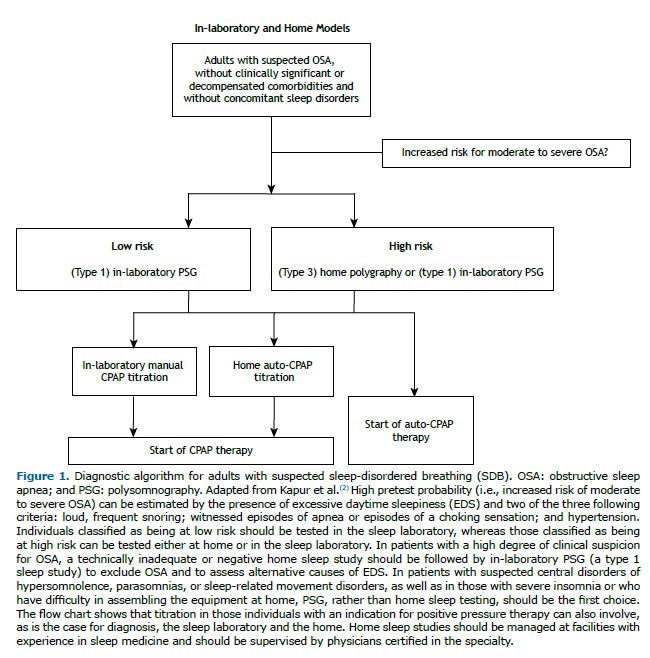
The home examination consists of measurements of airflow, respiratory effort, and SpO2. The sensors are applied by the patients themselves, following the instructions of a PSG technician or provided in an instructional video. Home devices must allow manual or automatic collection of data, which must always be reviewed by a specialist in sleep medicine and must always be read manually.(141,142) In comparison with a type 1 sleep study (attended PSG), type 3 (portable monitoring) sleep studies will typically underestimate the AHI, because they measure total recording time rather than total sleep time, as well as because they register only episodes of hypopnea that are accompanied by desaturation. This underestimation of the AHI by polygraphy does not seem to be clinically relevant. (143) However, it can be especially problematic for patients with mild OSA and for patients who frequently experience respiratory events unaccompanied by desaturation, including young people, women, and normal-weight individuals.
One systematic review evaluated validation studies of type 3 sleep studies versus type 1 sleep studies and found the accuracy of the former, in high-risk patients, to be as follows(2): 84-91% (AHI > 5 events/h); 65-91% (AHI > 15 events/h); and 81-94% (AHI > 30 events/h). Portable home methods showed excellent agreement and correlated well with the values obtained by complete, supervised PSG, especially in individuals with the more severe forms of OSA.(2) That same systematic review included studies aimed at comparing clinical outcomes when the diagnosis was made using a type 3 (portable monitoring) device versus a type 1 (PSG) device, and no differences were found in the EDS (assessed by the ESS), quality of life, or adherence to CPAP treatment. (2) However, it should be noted that, in that review,(2) the studies evaluated were limited to patients with a high probability of moderate-to-severe OSA, without significant comorbidities, and without other sleep disorders; in addition, they were conducted at centers with extensive experience in sleep medicine.
Due to their greater practicality and availability, portable devices allow more frequent reassessment of patients under treatment. Although routine reassessment is not indicated for all patients, those receiving treatments considered alternatives to CPAP, such as the use of a mandibular advancement device, positional therapy, surgery, or weight loss treatment, may benefit from a reassessment.(144)
High-resolution oximetry (a type 4 sleep study) detects cyclical oscillations in SpO2 that typically accompany respiratory events. Recent studies demonstrate a strong correlation between the PSG-derived AHI and the oxygen desaturation index, whether the latter is analyzed as a PSG-independent channel or through a high-resolution oximeter.(145-147) Nocturnal oximetry has clear advantages(2): it is an inexpensive, easily applied, noninvasive method; and there is robust evidence for its validity as a screening test for OSA, as well as in documenting the resolution of hypoxemia after treatment.(2) However, the method can produce false-negative results, cannot distinguish between OSA and central sleep apnea (CSA), and its accuracy can be reduced by various clinical factors, such as anemia, hypotension, peripheral vascular disorders, obesity, COPD, and frequent movements during sleep.(148,149) Therefore, further studies are needed in order to support the use of nocturnal home oximetry as a stand-alone diagnostic test for OSA, particularly in children and in individuals with significant comorbidities.(148)
The use of type 3 (portable monitoring) devices for the diagnosis of OSA in patients considered to be at high risk for moderate-to-severe OSA, without other suspected sleep disorders or significant cardiopulmonary or neurological comorbidities, is largely supported by validation studies and analyses of clinical outcomes. The use of such devices can reduce the huge demand for assessments in sleep laboratories.
Titration with positive pressure Positive pressure therapy is the treatment of choice for individuals with moderate-to-severe OSA.(150,151) The individualization of that therapy involves the determination of the ideal pressure to be applied in the UAs in order to control the obstructive respiratory events presented by the patient. This important part of positive pressure therapy can be performed in the sleep laboratory, under supervision (with the help of a full- or split-night PSG) or unsupervised at home.(150,151)
The gold standard for determining the optimal pressure to be applied in positive pressure therapy is manual titration during an overnight PSG in a sleep laboratory. (57,151,152) In that procedure, patients should receive educational information about the device, interfaces, beneficial effects, and the potential side effects of positive pressure therapy.The various interfaces (nasal mask, nasal pillow, and full face mask) and accessories (chin strap and heated humidifier) must be available on the night of the titration, and the patient must undergo desensitization, trying the interfaces connected to the device while it is turned on, before recording begins. This is an opportunity for patient education and due correction of any issues that may arise during the examination.(57) Nasal masks or nasal pillows should be considered the first choice of interface during titration because full face masks generally lead to a higher residual AHI, higher therapeutic pressure level, and greater leakage, which could reduce adherence to the treatment proposed. (153-156)
The manual titration protocols for CPAP and BiPAP follow the AASM algorithms,(152) as outlined in Figures 2 and 3.The titration is classified as optimal when there is complete control of respiratory events (AHI < 5 events/h and SpO2 ≥ 90%) for ≥ 15 min of REM sleep in the supine position. It is classified as good if the final respiratory disturbance index (RDI) is ≤ 10 or 50% lower than the baseline RDI if the latter was < 15 for ≥ 15 min of REM sleep in the supine position. It is classified as appropriate if the final RDI is not ≤ 10 but is ≥ 75% lower than the baseline RDI or if criteria for optimal or good titration are met but there was no REM sleep in the supine position. The titration is considered unacceptable if none of the criteria described above are met.(152)
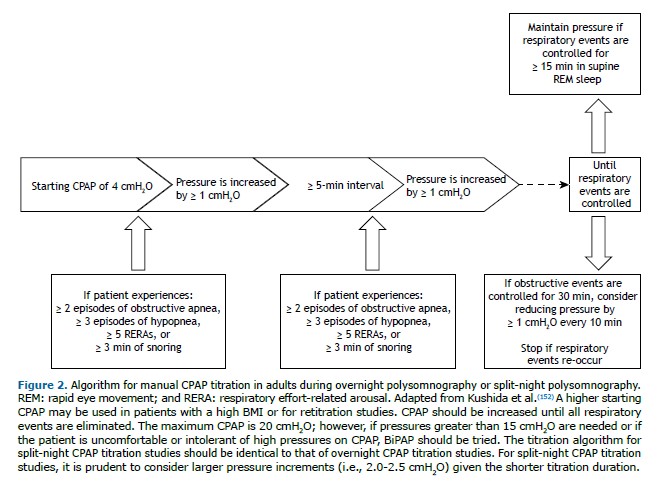
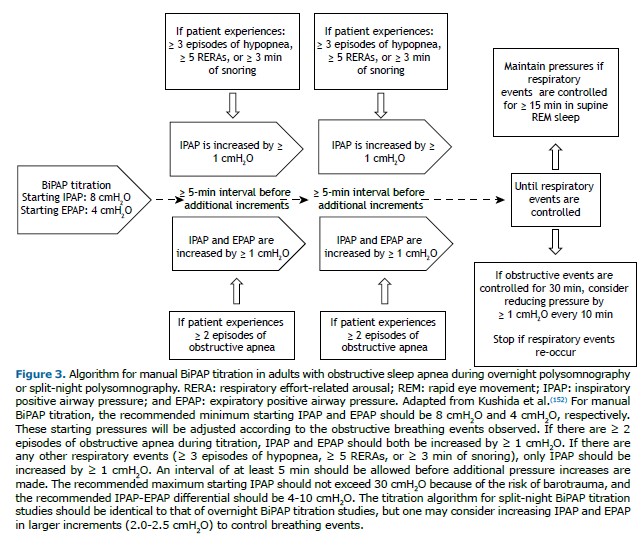
In patients presenting with hypoventilation or a history of hypoxemic lung disease, CPAP titration with supplemental oxygen may be necessary. Oxygen supplementation should be considered for patients who have a room-air SpO2 ≤ 88% while awake and in the supine position before titration. In addition, oxygen supplementation is used when the patient maintains a room-air SpO2 ≤ 88% for more than 5 min, even if there is complete control of obstructive events. Oxygen should be started at 1 L/min and titrated to maintain the SpO2 at 88-94%.(152)
During a CPAP titration study, some patients may develop treatment-emergent central sleep apnea (formerly known as complex sleep apnea syndrome), which is characterized by episodes of central sleep apnea in patients diagnosed with OSA.(157) In most cases, episodes of central apnea during an initial titration are transient and may disappear after continuous use of CPAP for 4-8 weeks, and it may be necessary to reduce the pressure level that was determined to be optimal during the titration.(158) When CPAP treatment fails to correct treatment-emergent central sleep apnea, it may be necessary to use adaptive servo-ventilation.(159)
Although the gold-standard practice involves manual titration of pressure during PSG performed in a sleep laboratory, that practice is laborious and costly. Therefore, the decision can be made to use auto-adjusting positive airway pressure (APAP) devices for automatic pressure titration, in a sleep laboratory or at home.(150,151) That decision should be based on access, cost, patient preference, and clinical judgment. Titration with automatic devices should not be performed in patients with hypoventilation, heart failure, COPD, neuromuscular disease, or nocturnal hypoxemia, as well as being inappropriate for use in non-snorers or in patients with CSA.(160) The potential benefits of home titration include its lower cost and faster initiation of treatment, due to easier access to the examination. For this type of titration, the patient must receive adequate educational guidance, problems must be quickly identified and corrected, and the physician must closely monitor the response to treatment.
Various studies have shown that there are no statistically significant differences between the mean treatment pressure obtained by home titration with APAP and that obtained by manual titration in a sleep laboratory.(110,161-163) A meta-analysis encompassing 10 randomized controlled trials compared the use of positive pressure using home titration with APAP and in-laboratory positive pressure titration(150): there were no clinically significant differences between the groups in adherence, sleepiness, or quality of life. A randomized clinical trial assessed patient preference for diagnostic/therapeutic management in the laboratory versus at home, showing that 62% of the participants randomized to in-laboratory management would have preferred home management, whereas only 6% of those randomized home management would have preferred in laboratory management.(164)
Positive pressure therapy Positive pressure therapy is currently recommended for patients with an AHI ≥ 15 events/h, as well as for those with an AHI of 5-14 events/h and EDS, cognitive impairment, mood disorders, insomnia, or coexisting conditions, such as hypertension, ischemic heart disease, and a history of stroke.(150,160) Although CPAP treatment significantly improves several clinical outcomes, adherence to the treatment is suboptimal in some cases.(165) Although CPAP adherence is defined as the use of CPAP for ≥ 4 h/night for at least 70% of the nights, there is a dose-response relationship between CPAP use and several clinical outcomes (Chart 10), a greater number of hours per night translating to a greater benefit.(53,55,166-170) Positive pressure devices provide pressure through a mask, preventing upper airway collapse and normalizing the AHI in more than 90% of users.(150) In a study analyzing data from more than 2.6 million patients initiating PAP therapy between 2014 and 2017, the aforementioned level of adherence was achieved by 75% within the first 90 days of treatment.(171) Of the patients who initiate PAP therapy, 65-80% are still using it 4 years later.(172,173) In a long-term follow-up study involving 107 patients with OSA, adequate adherence to PAP therapy (> 4 h/night) was observed in 57% of the patients in the first year, with no significant changes over the next 9 years.(174) Treatment adherence was found to correlate significantly with the severity of OSA (as assessed by the AHI) and ESS scores, although not with the pressure applied or the age of the patients.(174)
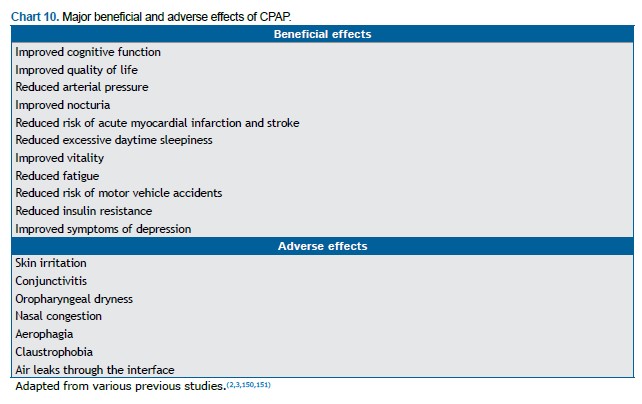
There are various approaches to positive pressure therapy, including BiPAP, APAP, and a reduction in pressure during the expiratory phase (expiratory pressure relief). In a meta-analysis of 23 randomized controlled trials, no clinically significant difference was observed between adults with OSA treated with APAP and those treated with CPAP regarding the mean number of hours of use.(151) Small clinical trials have shown that cognitive behavioral therapy or short-term use of a nonbenzodiazepine hypnotic can increase nocturnal CPAP use.(175,176) Patients with OSA who cannot tolerate positive pressure therapy are potential candidates for mandibular advancement devices, positional therapy (avoidance of the supine position during sleep), or surgery.(160)
In a randomized study comparing real and sham CPAP, real CPAP was found to improve vitality significantly in comparison with the sham treatment. (177) In a study comparing CPAP and placebo, fatigue and EDS were both found to be lower in the patients treated with CPAP.(178) Among patients with OSA, the risk of motor vehicle accidents has been reported to decrease substantially after CPAP treatment, becoming similar to that among drivers without OSA.(179) Treatment for OSA has a positive impact on cardiovascular health; in a study involving patients with an AHI ≥ 15 events/h, 24-h systolic blood pressure was found to be lower (by 4 mmHg) in those receiving CPAP than in those receiving placebo.(180) Similar results have been found in patients with resistant hypertension,(168) as well as in those with type 2 diabetes mellitus and hypertension.(181)
The effects that OSA treatment has on cardiovascular events remain unclear. In a randomized clinical trial comparing CPAP treatment with no treatment, involving patients without EDS and with an AHI ≥ 20 events/h, no appreciable reduction in a composite outcome of hypertension or cardiovascular events was observed over a 4-year period.(182) In a randomized study comparing CPAP with the standard of care among patients with established cardiovascular disease and moderate-to-severe OSA without severe sleepiness, CPAP was found to have no major effect on cardiovascular outcomes.(183) However, it should be noted that the two aforementioned studies had two major limitations: they excluded patients with EDS, which is an established risk factor for cardiovascular disease,(184) and adherence to CPAP treatment was low in their samples.
There are a number of factors that improve adherence to CPAP treatment(3): education regarding the risks of OSA and the expected benefits of positive pressure therapy; monitoring of CPAP use; and behavioral interventions, including cognitive behavioral therapy and motivational therapy. Technical solutions such as using humidifiers, automatic devices, and expiratory pressure relief devices to reduce side effects have shown no significant correlation with a increase in adherence to CPAP.(3,185,186)
Follow-up of patients receiving positive pressure therapy Patients undergoing CPAP treatment are usually monitored by a clinician reading the data stored in the CPAP device memory card. Those data provide information on three major parameters: adherence (number of hours of CPAP use per night and percentage of days using CPAP for > 4 h/night); air leaks; and residual AHI. Telemedicine use has increased dramatically, having an impact on the management (diagnosis, treatment, and follow-up) of SDB.(187-189) Telemonitoring of CPAP treatment involves the use of digital technologies to collect data on air leaks, residual AHI, and treatment adherence, which are electronically transmitted to the health care professional from the home of the patient.(190)
One of the main advantages of telemonitoring is early detection of treatment-related problems (air leaks or persistent respiratory events), facilitating appropriate interventions and improving the initial experience of patients with CPAP.(190,191) This is important because a favorable initial experience greatly contributes to long-term adherence.(150,151,192)
Telemonitoring appears to be well accepted by patients.(193) In addition, two meta-analyses showed that adherence to CPAP is significantly higher in telemonitoring groups than in standard monitoring groups.(194,195) In a study including a collective total of 4,181,490 patients in Brazil, Mexico, and the United States, more than 80% of the participants met the adherence criteria in the first 3 months (use of CPAP for ≥ 4 h/night for ≥ 70% of the nights), 1-year adherence rates being > 75%.(196)
Other treatments Treatment for OSA can include behavioral measures, oral appliances (OAs), and surgical procedures. Behavioral measures include avoiding the supine position, avoiding the use of alcohol especially before bedtime, engaging in regular aerobic exercise, and promoting weight loss. Avoiding the supine position reduces the risk of UA collapse. Positional therapy can involve the use of various accessories with different types of technology.(197) Weight loss improves OSA, and there is no minimum weight loss required to achieve this benefit; however, greater weight loss translates to a greater benefit.(198) Physical exercise can also improve OSA, independently of weight loss; although the mechanism has yet to be well understood, it appears to involve fat redistribution, fluid reabsorption, and increased pharyngeal muscle tone.(199)
The use of OAs for the treatment of OSA is an effective option, especially in patients with mild to moderate OSA. The appliances can also be used in patients with OSA who cannot tolerate CPAP. Although there are different types of OAs, the ones most commonly used are mandibular advancement devices that promote mandibular protrusion by altering the position of the tongue, thus increasing the UA diameter. Such appliances are effective in treating snoring in patients without OSA, as well as in reducing the AHI in patients with OSA,(200) leading to resolution of OSA in 60-80% of patients with mild OSA and in 30-50% of those with severe OSA.(201) However, it is highly unlikely that the 30-50% can be achieved in patients with severe OSA who are obese, are elderly, or have severe hypoxemia during sleep. Although CPAP has been shown to be more effective than are OAs in reducing the AHI and improving oxygenation, rates of treatment adherence can be higher for the latter.(202) Respiratory variables should be assessed in order to confirm the efficacy of treatment with OAs. Although the use of OAs can lead to changes in dental occlusion, it does not lead to significant skeletal changes. Their use can also cause temporary discomfort in the temporomandibular joint, masticatory muscles, or both, especially at the beginning of treatment. Side effects, including occlusal changes and pain, rarely lead to treatment discontinuation.(200,203)
Assessment and (clinical or surgical) treatment of nasal obstruction play an essential role in the management of OSA. Although nasal surgery alone does not have a consistent, significant effect of decreasing the AHI,(59) it can be used as an adjuvant treatment to improve adherence to CPAP.(204)
Surgical treatment is indicated for selected patients with OSA and is often recommended for those who cannot tolerate CPAP treatment.(205) The most commonly performed procedures involve removal of UA soft tissues and include uvulopalatopharyngoplasty, tongue base surgery, and lateral pharyngeal wall surgery. Because uvulopalatopharyngoplasty has a low success rate, new surgical techniques have been described and used, including lateral pharyngoplasty and expansion sphincter pharyngoplasty. However, there is no consensus regarding the best surgical technique and additional studies are needed in order to establish long-term efficacy.(205)
Maxillomandibular advancement surgery is indicated in specific cases of maxillomandibular disproportion, its reported rate of success—defined as a > 50% reduction in the AHI after surgery (to < 20 events/h)—being 85.5%.(206) Despite that high success rate, maxillomandibular advancement surgery is a major surgical procedure requiring postoperative follow-up. (206) In addition, because there are not enough studies confirming that it is effective, it should be recommended with caution and only after evaluation by a surgeon with experience in OSA.
Hypoglossal nerve stimulation is a recently described surgical procedure aimed at increasing pharyngeal dilator muscle tone.(207) However, the procedure has yet to be approved for use in Brazil and is not yet even available in the country. It consists in placing an electrode around the hypoglossal nerve, a sensor between the intercostal muscles to detect inspiratory effort, and a pulse generator in the chest wall. Hypoglossal nerve stimulation has been reported to lead to a significant decrease in the median AHI at 12 months (from 29.3 events/h to 9.0 events/h).(207) However, because it is a surgical procedure, appropriate patient selection is required, and drug-induced sleep endoscopy can aid in excluding the procedure as a treatment option.(208)
Bariatric surgery is the most effective and long-lasting treatment for obesity, reducing the risk of obesity-related comorbidities, as well as significantly reducing the number of obstructive respiratory events, improving oxygenation, and reducing mortality.(209-211) Among patients with class III obesity (BMI > 40 kg/m2) who undergo bariatric surgery, those with OSA appear to be at an increased risk of perioperative and postoperative complications.(212,213) Bariatric surgery effectively reduces body weight and significantly improves OSA, which can resolve completely in patients undergoing the procedure.(214,215)
Myofunctional therapy can also be used as an adjunct in the management of OSA.(216) This treatment modality consists of exercises targeting oral and oropharyngeal structures, and can lead to a reduction in the AHI in approximately 50% of patients with OSA.(216,217) In addition, it can improve oxygenation, snoring, and EDS,(216,217) and it can be used as an adjunct to other therapies.(217)
It is of note that the treatment of OSA is becoming more targeted to specific phenotypes, including compromised UA anatomy (UA narrowing/collapse), UA dilator muscle dysfunction, low arousal threshold, and unstable ventilatory control (high loop gain), with the objective of increasing the therapeutic response.(25,218) However, analysis of the aforementioned phenotypes is currently unfeasible in clinical practice, requiring specialized equipment and methods.(25,218)
HYPOVENTILATION SYNDROMES Hypoventilation is defined as an increase in PaCO2 to a level above the normal value of 45 mmHg in individuals at rest and awake.(219) Chart 11 shows the diseases that are most commonly associated with sleep hypoventilation. Although no single specific test can determine the cause of hypoventilation, the combined use of clinical assessment, physical examination, and ancillary tests (arterial blood gas analysis, pulmonary function tests, radiological evaluation, nocturnal oximetry, and PSG) can aid in identifying the etiology.(220)
Obesity hypoventilation syndrome Obesity hypoventilation syndrome (OHS) is defined as a combination of obesity (a BMI ≥ 30 kg/m2) and daytime PaCO2 > 45 mmHg at sea level in the absence of an alternative cause for hypercapnia, including lung disease, neuromuscular disease, metabolic disorders, and chest wall disease.(221-223) The AASM defines sleep hypoventilation as follows(123): PaCO2 (or transcutaneous/expired CO2 as a surrogate) > 55 mmHg for ≥ 10 min during sleep; or an increase in PaCO2 > 10 mmHg (in comparison with an awake supine value) to a value exceeding 50 mmHg for ≥ 10 min. In children, hypoventilation is defined as an increase in PaCO2 (or a surrogate) > 50 mmHg for > 25% of total sleep time.(123) Approximately 90% of patients with OHS also have OSA because obesity is a risk factor for both, the former also being known as hypercapnic OSA; in the 10% who do not have OSA, the sleep hypoventilation is not explained by episodes of apnea or hypopnea.(221-223)
Unlike patients with OSA alone, patients with OHS can present with dyspnea, leg edema, daytime hypoventilation, signs of cor pulmonale, and facial plethora,(221-223) as well as nocturnal OSA-related symptoms such as snoring, gasping/choking, and witnessed episodes of apnea.
An SpO2 < 93% and a venous serum bicarbonate concentration ≥ 27 mEq/L are suggestive of OHS,(223) and the differential diagnosis requires arterial blood gas analysis; pulmonary function testing and respiratory muscle strength (MIP and MEP) measurements; chest X-ray; electrocardiography; thyroid function testing; and PSG.
In patients with OHS, hypercapnia is multifactorial, including increased work of breathing; reduced central response to hypercapnia and hypoxemia; and reduced leptin activity. In comparison with other obese individuals, patients with OHS have decreased lung compliance, decreased functional residual capacity, and increased pulmonary resistance.(224)
The cornerstone of OHS treatment is weight loss, which can be achieved with bariatric surgery. Patients with OHS can benefit from CPAP treatment, which improves alveolar ventilation by reducing UA resistance, relieving respiratory muscle load, increasing central respiratory activity, or any combination of the three. Adult patients with OHS and concurrent severe OSA (AHI ≥ 30 events/h) presenting with stable chronic respiratory failure can be initially treated with CPAP rather than noninvasive ventilation (NIV).(222) It is of note that more than 70% of patients with OHS have severe OSA.(222) Therefore, this recommendation is applicable to the majority of patients with OHS. (222) However, there is less certainty in OHS patients without concomitant severe OSA.(222) Improvements in hypercapnia might be achieved more slowly with CPAP than with NIV during the initial weeks of treatment. (222) Patients with a higher degree of initial ventilatory failure, poorer lung function, advanced age, or OSA that is less severe might be less likely to respond to CPAP treatment.(222) Despite treatment adherence, hypercapnia and hypoxemia can persist in 20-50% of patients with OHS; in such cases, a switch to BiPAP is usually the next step.(224) Treatment with diuretics, medroxyprogesterone, acetazolamide, oxygen therapy alone, or tracheostomy is not currently recommended.(223)
Hypoventilation syndrome caused by neuromuscular disease Several neuromuscular diseases can cause respiratory muscle weakness, affecting children and adults (Chart 11). Although those diseases differ in their pathogenesis, treatment, and course, the final common pathway is respiratory muscle weakness resulting in alveolar hypoventilation.(220) Although PSG with capnography is essential for assessing sleep in patients with neuromuscular disease, a night in a sleep laboratory can be especially difficult for such patients, particularly if they require a personal care assistant. (225) The approach to these patients includes treating ventilatory muscle dysfunction, ineffective cough, and swallowing dysfunction, with the objective of protecting the airways.(225) The objective of NIV is to stabilize the decrease in vital capacity, to correct hypoxemia/hypercapnia, and to improve quality of life.(226) For patients with neuromuscular disease, NIV is considered the best treatment, and oxygen therapy (with NIV) should be provided only in cases in which NIV alone is unable to correct hypoxemia. The combined use of NIV and oxygen therapy leads to an improvement in survival in comparison with the use of oxygen therapy alone,(226,227) and these patients should not receive oxygen therapy without ventilatory support, because it could worsen hypoventilation.
Congenital central hypoventilation syndrome Congenital central hypoventilation syndrome (CCHS), also known as “Ondine’s curse”, is a rare condition characterized by disordered respiratory control. In addition to an abnormal respiratory drive, clinical manifestations include aganglionic megacolon, neural crest tumors, and autonomic nervous system abnormalities, including impaired heart rate control, impaired swallowing, gastroesophageal reflux, pupillary abnormalities, hypotonia, and profuse sweating.(219) The symptoms of CCHS typically appear in the first year of life, and diagnosis requires persistent evidence of hypoventilation in the absence of heart disease, lung disease, or neuromuscular disease.(219) Although CCHS primarily affects children, a small population of adults with mild, previously undetected CCHS has been identified.(220) The treatment of CCHS is based on ventilatory support.(228) Although NIV is ventilatory support of choice, electric stimulation of the diaphragm can also be used.(229,230)
Hypoventilation syndrome caused by lung disease Individuals with COPD are particularly prone to nocturnal hypoventilation, especially during REM sleep, due to loss of muscle tone and of accessory muscle function. In addition, many such patients show hyperinflation, which reduces diaphragmatic efficiency. Hypoventilation is exacerbated by reduced ventilatory responsiveness to CO2 during sleep, resulting in nocturnal oxygen desaturation and predisposing to pulmonary hypertension, cardiac arrhythmias, and reduced sleep quality, as well as being a marker of increased mortality.
In patients with COPD, resting daytime hypoxemia, and evidence of right ventricular dysfunction, oxygen therapy reduces mortality, reduces exacerbations, and improves quality of life, a greater number of hours of use translating to a greater benefit.(89,231,232) There is no consensus as to whether patients with nocturnal oxygen desaturation alone should use oxygen therapy or NIV.(89,231,232)
Patients with overlap syndrome have more significant nocturnal hypoventilation, as well as greater hypoxemia and hypercapnia, than do those with COPD or OSA alone.(231) The standard treatment for overlap syndrome is CPAP because it relieves UA obstruction, relieves the respiratory muscles, reduces hypoventilation, reduces oxygen consumption, and reduces CO2 production by the respiratory muscles.(232) Because CPAP alone might not be able to correct hypoxemia fully, oxygen therapy is also required.(231,232)
CENTRAL SLEEP APNEA It is known that CSA is much less common than is OSA.(233) Central apnea is the cessation of airflow with no respiratory effort; patients with an AHI ≥ 5 events/h, more than 50% of which are central respiratory events, are classified as having CSA.(233,234) Home polygraphs have yet to be validated for use in the diagnosis of CSA, PSG therefore continuing to be the gold standard.(234)
On the basis of its pathophysiology, CSA can be divided into two groups(235): eucapnic CSA (the more common of the two, being accompanied by Cheyne-Stokes respiration and affecting patients with heart failure, acute ischemic stroke, and periodic breathing at high altitude); and hypercapnic CSA (which is observed in individuals with neuromuscular disease, rib cage abnormalities, or opioid intoxication). Hypercapnic CSA arises from changes in the respiratory center, chemoreceptors, or skeletal muscles, leading to hypoventilation.(235,236) In contrast, eucapnic CSA is caused by instability in the regulatory pathways that control ventilation(235,236): disturbances such as hypoxemia or transitions from wakefulness to sleep are associated with periods of hyperventilation, which lead to increased minute ventilation and subsequent decreases in PaCO2. When PaCO2 falls below the apnea threshold, as determined by the central chemoreceptors, a central apnea occurs.(235,236)
Heart failure is perhaps the condition that is most commonly associated with CSA (or, more specifically, with Cheyne-Stokes respiration).(234) In a cross-sectional analysis of the Sleep Heart Health study, the overall prevalence of CSA in patients with heart failure was found to be 0.9%, and approximately half of the patients with CSA also had Cheyne-Stokes respiration.(237) Although Cheyne-Stokes respiration can occur during wakefulness and during sleep, it is more common during the latter: when Cheyne-Stokes respiration occurs during sleep, it is a form of CSA with prolonged hyperpnea and denotes low cardiac output.(234) The diagnostic criteria for Cheyne-Stokes respiration include at least three consecutive episodes of central apnea or hypopnea separated by crescendo-decrescendo respirations with a cycle length of at least 40 s.(234)
Initial population-based studies reported that approximately 40% of men with systolic heart failure had CSA, and subsequent studies, some of which also included women, showed similar proportions. (238,239) Central events occur most frequently during the lighter stages of non-REM sleep, particularly after arousals and sleep stage changes.(238)
A form of Cheyne-Stokes respiration can also be observed during periodic breathing at high altitude. Altered breathing during non-REM sleep commonly occurs in this situation because of changes in neural signaling caused by hypoxia (attributed to a decrease in FiO2) and hyperventilation-induced alkalosis. Although this can occur at elevations as low as 1,400 m, it rarely causes symptoms at elevations below 2,500 m.(240)
Although stroke has been considered a risk factor for CSA, an analysis of central and obstructive events in patients with stroke shows that OSA is much more common than is CSA in such patients.(241,242) Opioid intoxication is another risk factor for CSA because opioids decrease central and peripheral responsiveness to hypoxemia and hypercapnia during wakefulness and sleep.(243) Although the exact mechanism of CSA caused by chronic opioid use has yet to be understood, it might be partly due to a change in respiratory drive caused by reduced chemoreceptor sensitivity. The most common sleep-related disorder in chronic opioid users is CSA because although chronic opioid use can lead to worsening of obstructive events, it plays a greater role in the development of central events.(244)
The mainstay of the treatment of hypercapnic and eucapnic CSA is optimization in relation to the underlying cause.(234) For patients with an AHI > 15 events/h (with a predominance of central events) and significant symptoms, CPAP can be used.(245) If a decision is made to initiate positive pressure therapy, CPAP is the preferred choice because it has led to improvements in patients with heart failure. (246,247) The addition of oxygen therapy is indicated if PSG demonstrates episodes of nocturnal desaturation associated with central events.(234) If symptoms persist despite initiation of CPAP treatment or if patients cannot tolerate that treatment, other positive pressure modes can be used.(234) The most important determining factor of which mode to use in the treatment of CSA associated with heart failure is the ejection fraction; in patients with an ejection fraction < 45%, it remains unclear what the optimal treatment is. One study(248) showed that adaptive servo-ventilation should not be used in patients with an ejection fraction ≤ 45%, because, in comparison with guideline-based medical treatment alone, it was found to increase all-cause mortality (35% vs. 29%; hazard ratio, 1.28; 95% CI: 1.06-1.55) and cardiovascular mortality (30% vs. 24%; hazard ratio, 1.34; 95% CI: 1.09-1.65).
Oxygen therapy (if needed) combined with optimization of heart failure treatment can be the optimal approach for patients with CSA.(234) Adaptive servo-ventilation and BiPAP with a backup rate are available treatment options for patients who have an ejection fraction > 45% and who cannot tolerate CPAP treatment.(234) It has recently been shown that phrenic nerve stimulators ensure stable ventilation, improving physical performance and reducing hypoxemia.(249)
OBSTRUCTIVE SLEEP APNEA IN CHILDREN In children, SDB ranges from primary snoring to OSA (Chart 12).(250) The estimated overall prevalence of OSA in children (of any age) is 1-4%, being significantly higher in obese children.(251) The incidence of OSA in children peaks between 2 and 8 years of age, which is the age range in which the pharyngeal and palatine tonsils are largest in relation to the UAs. It tends to be more common in children with a family history of OSA, in those of African or Asian descent, and in those with clinical conditions that lead to UA obstruction; those with craniofacial abnormalities; and those with lysosomal storage disorders.(251,252)
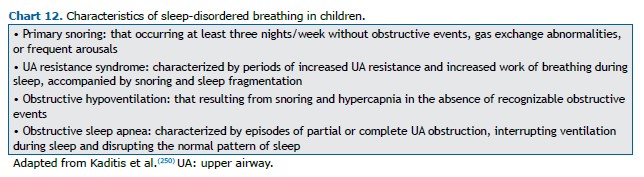
The pathophysiology of OSA in children is similar to that of OSA in adults in that UA narrowing occurs during sleep as a result of decreased muscle tone and, consequently, increased UA resistance caused by loss of the wakefulness stimuli. In pediatric patients, OSA is probably due to a combination of changes in UA structure and reduced neuromuscular control, together with genetic, hormonal, inflammatory, metabolic, and environmental factors (exposure to smoking and environmental pollution).(252,253)
The main predictors of OSA are snoring, witnessed episodes of apnea, and restless sleep. Screening for OSA in children should include questions regarding the following(250,254): snoring (frequency, intensity, body position, persistence, and association with respiratory effort); behavior during sleep and upon waking; sleep position; enuresis; recurrent UA infections; mouth breathing; school performance; emotional lability; and comorbidities.
In the physical examination of children with suspected OSA, the following aspects should be investigated(253): height and weight (because children with OSA tend to present with growth impairment and weigh less than expected for their age); evidence of chronic UA obstruction (signs of mouth breathing); enlarged palatine tonsils (in the transverse and anteroposterior diameters); craniofacial features (long, narrow facial features, receding chin, retrognathia, high-arched palate, elongated soft palate, and malocclusion); and signs of right ventricular overload and hypertension. Because the prevalence of OSA is high in patients with syndromes, neuromuscular disorders, and craniofacial (skeletal or soft-tissue) abnormalities, such patients should be carefully evaluated.(250)
For the diagnosis of OSA and the monitoring of patients under treatment for OSA, the standard test is PSG performed in a sleep laboratory. Infants ≤ 6 months of age can undergo daytime PSG (the recommended recording time being 3-4 h). Unlike adult OSA, pediatric OSA can be diagnosed on the basis of 1 event/h of sleep, 1.5 events/h of sleep, or 2 events/h of sleep. An obstructive AHI > 5 events/h indicates moderate OSA, and an obstructive AHI ≥ 10 events/h indicates severe OSA.(250) In adolescents, OSA is diagnosed on the basis of the same criteria as those used for the diagnosis of OSA in adults because specific criteria have yet to be established. (255) Evaluation of CO2 during PSG, by capnography or transcutaneous monitoring, plays an important role in identifying hypoventilation in children.(133) Although CO2 measurement is highly recommended, it is not currently widely available in Brazil because there is a lack of CO2 measurement devices approved for use by the Brazilian National Health Regulatory Agency. Although home sleep monitoring remains controversial, it has been used in settings in which access to PSG is limited and the results have been promising.(250)
Pediatric PSG should be performed in the following situations(250): in children with symptoms of obstructive SDB before undergoing adenotonsillectomy, particularly in those with obesity, craniofacial abnormalities, neuromuscular disorders, or complex abnormalities (e.g., Chiari malformation, Down syndrome, and Prader-Willi syndrome), as well as in those in whom the need for treatment is unclear; in patients with persistent symptoms of OSA despite having undergone adenotonsillectomy; in those presenting with moderate-to-severe OSA in the preoperative period; and in those presenting with obesity, craniofacial abnormalities, neuromuscular disorders, or complex abnormalities (e.g., Chiari malformation, Down syndrome, and Prader-Willi syndrome); and before and after OSA treatment with rapid maxillary expansion, OAs, CPAP, or NIV.
Ancillary tests such as fiberoptic laryngoscopy, cephalometry, CT of the facial bones followed by three-dimensional reconstruction of the images, spirometry, and arterial blood gas analysis can be used depending on the clinical context. In patients with severe abnormalities who are candidates for surgery, echocardiography for assessment of the right heart and detection of pulmonary hypertension can be indicated.
Surgery (adenotonsillectomy) is still the treatment of first choice for patients with lymphoid tissue hypertrophy. (253) However, surgical treatment is more likely to fail or only partially succeed in infants, obese children, patients with genetic disorders that result in craniofacial abnormalities, and patients with severe OSA.(252)
Postoperative respiratory complications have been reported to be common in children with severe OSA, those < 3 years of age, and those with comorbidities. Such children should be continuously and noninvasively monitored for oxygen and CO2 levels, preferably in the ICU.(253) Surgical procedures such as mandibular distraction osteogenesis, maxillomandibular advancement, and, in extreme cases, tracheostomy can be performed, especially in patients with craniofacial abnormalities. At some referral centers, bariatric surgery has been performed, albeit rarely, in obese adolescents.
In individuals with OSA, other treatment approaches can be used in isolation or in combination with adenotonsillectomy, including the use of topical nasal corticosteroids or leukotriene receptor antagonists; weight loss diets; orofacial myofunctional therapy; orthodontic and dentofacial orthopedic treatment; and CPAP. Older children and adolescents with OSA can have clinical characteristics that are similar to those of adults, the recommended therapeutic approach therefore being similar.(57)
There is increasing evidence of the cardiac impact of inadequately treated OSA, including hypertension, tachycardia, increased heart rate variability, right ventricular dysfunction, and pulmonary hypertension. Great emphasis has been given to neurocognitive impairments, with evidence that OSA can affect mood, language skills, visual perception, and memory.(252) The most significant neurocognitive and cardiovascular sequelae are described in Chart 13.
AUTHOR CONTRIBUTIONS All of the authors contributed to the conceptualization and planning of the study, as well as to the writing, reviewing, and revising of the manuscript, having approved the final version before submission.
CONFLICTS OF INTEREST None declared.
REFERENCES 1. Ramar K, Malhotra RK, Carden KA, Martin JL, Abbasi-Feinberg F, Aurora RN, et al. Sleep is essential to health: an American Academy of Sleep Medicine position statement. J Clin Sleep Med. 2021;17(10):2115-2119. https://doi.org/10.5664/jcsm.9476
2. Kapur VK, Auckley DH, Chowdhuri S, Kuhlmann DC, Mehra R, Ramar K, et al. Clinical Practice Guideline for Diagnostic Testing for Adult Obstructive Sleep Apnea: An American Academy of Sleep Medicine Clinical Practice Guideline. J Clin Sleep Med. 2017;13(3):479-504. https://doi.org/10.5664/jcsm.6506
3. Gottlieb DJ, Punjabi NM. Diagnosis and Management of Obstructive Sleep Apnea: A Review. JAMA. 2020;323(14):1389-1400. https://doi.org/10.1001/jama.2020.3514
4. Dodds S, Williams LJ, Roguski A, Vennelle M, Douglas NJ, Kotoulas SC, et al. Mortality and morbidity in obstructive sleep apnoea-hypopnoea syndrome: results from a 30-year prospective cohort study. ERJ Open Res. 2020;6(3):00057-2020. https://doi.org/10.1183/23120541.00057-2020
5. Huyett P, Bhattacharyya N. Incremental health care utilization and expenditures for sleep disorders in the United States. J Clin Sleep Med. 2021;17(10):1981-1986. https://doi.org/10.5664/jcsm.9392
6. Lyons MM, Bhatt NY, Pack AI, Magalang UJ. Global burden of sleep-disordered breathing and its implications. Respirology. 2020;25(7):690-702. https://doi.org/10.1111/resp.13838
7. Young T, Palta M, Dempsey J, Skatrud J, Weber S, Badr S. The occurrence of sleep-disordered breathing among middle-aged adults. N Engl J Med. 1993;328(17):1230-1235. https://doi.org/10.1056/NEJM199304293281704
8. Peppard PE, Young T, Barnet JH, Palta M, Hagen EW, Hla KM. Increased prevalence of sleep-disordered breathing in adults. Am J Epidemiol. 2013;177(9):1006-1014. https://doi.org/10.1093/aje/kws342
9. Heinzer R, Vat S, Marques-Vidal P, Marti-Soler H, Andries D, Tobback N, et al. Prevalence of sleep-disordered breathing in the general population: the HypnoLaus study. Lancet Respir Med. 2015;3(4):310-318. https://doi.org/10.1016/S2213-2600(15)00043-0
10. Fietze I, Laharnar N, Obst A, Ewert R, Felix SB, Garcia C, et al. Prevalence and association analysis of obstructive sleep apnea with gender and age differences - Results of SHIP-Trend. J Sleep Res. 2019;28(5):e12770. https://doi.org/10.1111/jsr.12770
11. Tufik S, Santos-Silva R, Taddei JA, Bittencourt LR. Obstructive sleep apnea syndrome in the Sao Paulo Epidemiologic Sleep Study. Sleep Med. 2010;11(5):441-446. https://doi.org/10.1016/j.sleep.2009.10.005
12. Senaratna CV, Perret JL, Lodge CJ, Lowe AJ, Campbell BE, Matheson MC, et al. Prevalence of obstructive sleep apnea in the general population: A systematic review. Sleep Med Rev. 2017;34:70-81. https://doi.org/10.1016/j.smrv.2016.07.002
13. Lam B, Ip MS, Tench E, Ryan CF. Craniofacial profile in Asian and white subjects with obstructive sleep apnoea. Thorax. 2005;60(6):504-510. https://doi.org/10.1136/thx.2004.031591
14. Benjafield AV, Ayas NT, Eastwood PR, Heinzer R, Ip MSM, Morrell MJ, et al. Estimation of the global prevalence and burden of obstructive sleep apnoea: a literature-based analysis. Lancet Respir Med. 2019;7(8):687-698. https://doi.org/10.1016/S2213-2600(19)30198-5
15. Kositanurit W, Muntham D, Udomsawaengsup S, Chirakalwasan N. Prevalence and associated factors of obstructive sleep apnea in morbidly obese patients undergoing bariatric surgery. Sleep Breath. 2018;22(1):251-256. https://doi.org/10.1007/s11325-017-1500-y
16. Ravesloot MJ, van Maanen JP, Hilgevoord AA, van Wagensveld BA, de Vries N. Obstructive sleep apnea is underrecognized and underdiagnosed in patients undergoing bariatric surgery. Eur Arch Otorhinolaryngol. 2012;269(7):1865-1871. https://doi.org/10.1007/s00405-012-1948-0
17. Muxfeldt ES, Margallo VS, Guimarães GM, Salles GF. Prevalence and associated factors of obstructive sleep apnea in patients with resistant hypertension. Am J Hypertens. 2014;27(8):1069-1078. https://doi.org/10.1093/ajh/hpu023
18. Pedrosa RP, Drager LF, Gonzaga CC, Sousa MG, de Paula LK, Amaro AC, et al. Obstructive sleep apnea: the most common secondary cause of hypertension associated with resistant hypertension. Hypertension. 2011;58(5):811-817. https://doi.org/10.1161/HYPERTENSIONAHA.111.179788
19. Youssef I, Kamran H, Yacoub M, Patel N, Goulbourne C, Kumar S, et al. Obstructive Sleep Apnea as a Risk Factor for Atrial Fibrillation: A Meta-Analysis. J Sleep Disord Ther. 2018;7(1):282. https://doi.org/10.4172/2167-0277.1000282
20. Li M, Hou WS, Zhang XW, Tang ZY. Obstructive sleep apnea and risk of stroke: a meta-analysis of prospective studies. Int J Cardiol. 2014;172(2):466-469. https://doi.org/10.1016/j.ijcard.2013.12.230
21. Zhang Y, Ren R, Lei F, Zhou J, Zhang J, Wing YK, et al. Worldwide and regional prevalence rates of co-occurrence of insomnia and insomnia symptoms with obstructive sleep apnea: A systematic review and meta-analysis. Sleep Med Rev. 2019;45:1-17. https://doi.org/10.1016/j.smrv.2019.01.004
22. Luyster FS, Buysse DJ, Strollo PJ Jr. Comorbid insomnia and obstructive sleep apnea: challenges for clinical practice and research. J Clin Sleep Med. 2010;6(2):196-204. https://doi.org/10.5664/jcsm.27772
23. Lechat B, Appleton S, Melaku YA, Hansen K, McEvoy RD, Adams R, et al. Co-morbid insomnia and obstructive sleep apnoea is associated with all-cause mortality. Eur Respir J. 2021;2101958. https://doi.org/10.1183/13993003.01958-2021
24. Eckert DJ, Malhotra A. Pathophysiology of adult obstructive sleep apnea. Proc Am Thorac Soc. 2008;5(2):144-153. https://doi.org/10.1513/pats.200707-114MG
25. Eckert DJ. Phenotypic approaches to obstructive sleep apnoea - New pathways for targeted therapy. Sleep Med Rev. 2018;37:45-59. https://doi.org/10.1016/j.smrv.2016.12.003
26. Taranto-Montemurro L, Messineo L, Wellman A. Targeting Endotypic Traits with Medications for the Pharmacological Treatment of Obstructive Sleep Apnea. A Review of the Current Literature. J Clin Med. 2019;8(11):1846. https://doi.org/10.3390/jcm8111846
27. Somers VK, Dyken ME, Mark AL, Abboud FM. Sympathetic-nerve activity during sleep in normal subjects. N Engl J Med. 1993;328(5):303-307. https://doi.org/10.1056/NEJM199302043280502
28. Kim AM, Keenan BT, Jackson N, Chan EL, Staley B, Poptani H, et al. Tongue fat and its relationship to obstructive sleep apnea. Sleep. 2014;37(10):1639-1648. https://doi.org/10.5665/sleep.4072
29. Peppard PE, Young T, Palta M, Dempsey J, Skatrud J. Longitudinal study of moderate weight change and sleep-disordered breathing. JAMA. 2000;284(23):3015-3021. https://doi.org/10.1001/jama.284.23.3015
30. Stanchina ML, Malhotra A, Fogel RB, Trinder J, Edwards JK, Schory K, et al. The influence of lung volume on pharyngeal mechanics, collapsibility, and genioglossus muscle activation during sleep. Sleep. 2003;26(7):851-856. https://doi.org/10.1093/sleep/26.7.851
31. Kawaguchi Y, Fukumoto S, Inaba M, Koyama H, Shoji T, Shoji S, et al. Different impacts of neck circumference and visceral obesity on the severity of obstructive sleep apnea syndrome. Obesity (Silver Spring). 2011;19(2):276-282. https://doi.org/10.1038/oby.2010.170
32. Dancey DR, Hanly PJ, Soong C, Lee B, Shepard J Jr, Hoffstein V. Gender differences in sleep apnea: the role of neck circumference. Chest. 2003;123(5):1544-1550. https://doi.org/10.1378/chest.123.5.1544
33. Onat A, Hergenç G, Yüksel H, Can G, Ayhan E, Kaya Z, et al. Neck circumference as a measure of central obesity: associations with metabolic syndrome and obstructive sleep apnea syndrome beyond waist circumference. Clin Nutr. 2009;28(1):46-51. https://doi.org/10.1016/j.clnu.2008.10.006
34. Shah N, Hanna DB, Teng Y, Sotres-Alvarez D, Hall M, Loredo JS, et al. Sex-Specific Prediction Models for Sleep Apnea From the Hispanic Community Health Study/Study of Latinos. Chest. 2016;149(6):1409-1418. https://doi.org/10.1016/j.chest.2016.01.013
35. Redolfi S, Yumino D, Ruttanaumpawan P, Yau B, Su MC, Lam J, et al. Relationship between overnight rostral fluid shift and Obstructive Sleep Apnea in nonobese men. Am J Respir Crit Care Med. 2009;179(3):241-246. https://doi.org/10.1164/rccm.200807-1076OC
36. Bixler EO, Vgontzas AN, Lin HM, Ten Have T, Rein J, Vela-Bueno A, et al. Prevalence of sleep-disordered breathing in women: effects of gender. Am J Respir Crit Care Med. 2001;163(3 Pt 1):608-613. https://doi.org/10.1164/ajrccm.163.3.9911064
37. Mirer AG, Young T, Palta M, Benca RM, Rasmuson A, Peppard PE. Sleep-disordered breathing and the menopausal transition among participants in the Sleep in Midlife Women Study. Menopause. 2017;24(2):157-162. https://doi.org/10.1097/GME.0000000000000744
38. Malhotra A, Huang Y, Fogel RB, Pillar G, Edwards JK, Kikinis R, et al. The male predisposition to pharyngeal collapse: importance of airway length. Am J Respir Crit Care Med. 2002;166(10):1388-1395. https://doi.org/10.1164/rccm.2112072
39. Jordan AS, McEvoy RD. Gender differences in sleep apnea: epidemiology, clinical presentation and pathogenic mechanisms. Sleep Med Rev. 2003;7(5):377-389. https://doi.org/10.1053/smrv.2002.0260
40. Caufriez A, Leproult R, L’Hermite-Balériaux M, Kerkhofs M, Copinschi G. Progesterone prevents sleep disturbances and modulates GH, TSH, and melatonin secretion in postmenopausal women. J Clin Endocrinol Metab. 2011;96(4):E614-E623. https://doi.org/10.1210/jc.2010-2558
41. Lin TY, Lin PY, Su TP, Li CT, Lin WC, Chang WH, et al. Risk of developing obstructive sleep apnea among women with polycystic ovarian syndrome: a nationwide longitudinal follow-up study. Sleep Med. 2017;36:165-169. https://doi.org/10.1016/j.sleep.2016.12.029
42. Liu PY, Yee B, Wishart SM, Jimenez M, Jung DG, Grunstein RR, et al. The short-term effects of high-dose testosterone on sleep, breathing, and function in older men. J Clin Endocrinol Metab. 2003;88(8):3605-3613. https://doi.org/10.1210/jc.2003-030236
43. Ernst G, Mariani J, Blanco M, Finn B, Salvado A, Borsini E. Increase in the frequency of obstructive sleep apnea in elderly people. Sleep Sci. 2019;12(3):222-226. https://doi.org/10.5935/1984-0063.20190081
44. Malhotra A, Huang Y, Fogel R, Lazic S, Pillar G, Jakab M, et al. Aging influences on pharyngeal anatomy and physiology: the predisposition to pharyngeal collapse. Am J Med. 2006;119(1):72.e9-72.e7.2E14. https://doi.org/10.1016/j.amjmed.2005.01.077
45. Gooneratne NS, Vitiello MV. Sleep in older adults: normative changes, sleep disorders, and treatment options. Clin Geriatr Med. 2014;30(3):591-627. https://doi.org/10.1016/j.cger.2014.04.007
46. Arnaud C, Bochaton T, Pépin JL, Belaidi E. Obstructive sleep apnoea and cardiovascular consequences: Pathophysiological mechanisms. Arch Cardiovasc Dis. 2020;113(5):350-358. https://doi.org/10.1016/j.acvd.2020.01.003
47. Reutrakul S, Mokhlesi B. Obstructive Sleep Apnea and Diabetes: A State of the Art Review. Chest. 2017;152(5):1070-1086. https://doi.org/10.1016/j.chest.2017.05.009
48. Li M, Li X, Lu Y. Obstructive Sleep Apnea Syndrome and Metabolic Diseases. Endocrinology. 2018;159(7):2670-2675. https://doi.org/10.1210/en.2018-00248
49. Dredla BK, Castillo PR. Cardiovascular Consequences of Obstructive Sleep Apnea. Curr Cardiol Rep. 2019;21(11):137. https://doi.org/10.1007/s11886-019-1228-3
50. Salman LA, Shulman R, Cohen JB. Obstructive Sleep Apnea, Hypertension, and Cardiovascular Risk: Epidemiology, Pathophysiology, and Management. Curr Cardiol Rep. 2020;22(2):6. https://doi.org/10.1007/s11886-020-1257-y
51. Yaggi HK, Concato J, Kernan WN, Lichtman JH, Brass LM, Mohsenin V. Obstructive sleep apnea as a risk factor for stroke and death. N Engl J Med. 2005;353(19):2034-2041. https://doi.org/10.1056/NEJMoa043104
52. Lee CH, Sethi R, Li R, Ho HH, Hein T, Jim MH, et al. Obstructive Sleep Apnea and Cardiovascular Events After Percutaneous Coronary Intervention. Circulation. 2016;133(21):2008-2017. https://doi.org/10.1161/CIRCULATIONAHA.115.019392
53. Marin JM, Carrizo SJ, Vicente E, Agusti AG. Long-term cardiovascular outcomes in men with obstructive sleep apnoea-hypopnoea with or without treatment with continuous positive airway pressure: an observational study. Lancet. 2005;365(9464):1046-1053. https://doi.org/10.1016/S0140-6736(05)71141-7
54. Campos-Rodriguez F, Martinez-Garcia MA, de la Cruz-Moron I, Almeida-Gonzalez C, Catalan-Serra P, Montserrat JM. Cardiovascular mortality in women with obstructive sleep apnea with or without continuous positive airway pressure treatment: a cohort study. Ann Intern Med. 2012;156(2):115-122. https://doi.org/10.7326/0003-4819-156-2-201201170-00006
55. Martínez-García MA, Campos-Rodríguez F, Catalán-Serra P, Soler-Cataluña JJ, Almeida-Gonzalez C, De la Cruz Morón I, et al. Cardiovascular mortality in obstructive sleep apnea in the elderly: role of long-term continuous positive airway pressure treatment: a prospective observational study. Am J Respir Crit Care Med. 2012;186(9):909-916. https://doi.org/10.1164/rccm.201203-0448OC
56. Mehra R. Sleep apnea and the heart. Cleve Clin J Med. 2019;86(9 Suppl 1):10-18. https://doi.org/10.3949/ccjm.86.s1.03
57. Epstein LJ, Kristo D, Strollo PJ Jr, Friedman N, Malhotra A, Patil SP, et al. Clinical guideline for the evaluation, management and long-term care of obstructive sleep apnea in adults. J Clin Sleep Med. 2009;5(3):263-276. https://doi.org/10.5664/jcsm.27497
58. Carnethon MR, Johnson DA. Sleep and Resistant Hypertension. Curr Hypertens Rep. 2019;21(5):34. https://doi.org/10.1007/s11906-019-0941-z
59. Van Ryswyk E, Mukherjee S, Chai-Coetzer CL, Vakulin A, McEvoy RD. Sleep Disorders, Including Sleep Apnea and Hypertension. Am J Hypertens. 2018;31(8):857-864. https://doi.org/10.1093/ajh/hpy082
60. Cai A, Wang L, Zhou Y. Hypertension and obstructive sleep apnea. Hypertens Res. 2016;39(6):391-395. https://doi.org/10.1038/hr.2016.11
61. Lyons OD, Ryan CM. Sleep Apnea and Stroke. Can J Cardiol. 2015;31(7):918-927. https://doi.org/10.1016/j.cjca.2015.03.014
62. Arzt M, Young T, Finn L, Skatrud JB, Bradley TD. Association of sleep-disordered breathing and the occurrence of stroke. Am J Respir Crit Care Med. 2005;172(11):1447-1451. https://doi.org/10.1164/rccm.200505-702OC
63. McKee Z, Auckley DH. A sleeping beast: Obstructive sleep apnea and stroke. Cleve Clin J Med. 2019;86(6):407-415. https://doi.org/10.3949/ccjm.86a.18033
64. Drager LF, Togeiro SM, Polotsky VY, Lorenzi-Filho G. Obstructive sleep apnea: a cardiometabolic risk in obesity and the metabolic syndrome. J Am Coll Cardiol. 2013;62(7):569-576. https://doi.org/10.1016/j.jacc.2013.05.045
65. Gaines J, Vgontzas AN, Fernandez-Mendoza J, Bixler EO. Obstructive sleep apnea and the metabolic syndrome: The road to clinically-meaningful phenotyping, improved prognosis, and personalized treatment. Sleep Med Rev. 2018;42:211-219. https://doi.org/10.1016/j.smrv.2018.08.009
66. Hirotsu C, Haba-Rubio J, Togeiro SM, Marques-Vidal P, Drager LF, Vollenweider P, et al. Obstructive sleep apnoea as a risk factor for incident metabolic syndrome: a joined Episono and HypnoLaus prospective cohorts study. Eur Respir J. 2018;52(5):1801150. https://doi.org/10.1183/13993003.01150-2018
67. Dorkova Z, Petrasova D, Molcanyiova A, Popovnakova M, Tkacova R. Effects of continuous positive airway pressure on cardiovascular risk profile in patients with severe obstructive sleep apnea and metabolic syndrome. Chest. 2008;134(4):686-692. https://doi.org/10.1378/chest.08-0556
68. Giampá SQC, Furlan SF, Freitas LS, Macedo TA, Lebkuchen A, Cardozo KHM, et al. Effects of CPAP on Metabolic Syndrome in Patients With OSA: A Randomized Trial. Chest. 2022;161(5):1370-1381. https://doi.org/10.1016/j.chest.2021.12.669
69. Chaudhary P, Goyal A, Pakhare A, Goel SK, Kumar A, Reddy MA, et al. Metabolic syndrome in non-obese patients with OSA: learning points of a cross-sectional study from a tertiary care hospital in Central India. Sleep Breath. 2022;26(2):681-688. https://doi.org/10.1007/s11325-021-02401-4
70. Yu Z, Cheng JX, Zhang D, Yi F, Ji Q. Association between Obstructive Sleep Apnea and Type 2 Diabetes Mellitus: A Dose-Response Meta-Analysis. Evid Based Complement Alternat Med. 2021;2021:1337118. https://doi.org/10.1155/2021/1337118
71. Panossian LA, Veasey SC. Daytime sleepiness in obesity: mechanisms beyond obstructive sleep apnea--a review. Sleep. 2012;35(5):605-615. https://doi.org/10.5665/sleep.1812
72. Chapman JL, Serinel Y, Marshall NS, Grunstein RR. Residual Daytime Sleepiness in Obstructive Sleep Apnea After Continuous Positive Airway Pressure Optimization: Causes and Management. Sleep Med Clin. 2016;11(3):353-363. https://doi.org/10.1016/j.jsmc.2016.05.005
73. Vanek J, Prasko J, Genzor S, Ociskova M, Kantor K, Holubova M, et al. Obstructive sleep apnea, depression and cognitive impairment. Sleep Med. 2020;72:50-58. https://doi.org/10.1016/j.sleep.2020.03.017
74. BaHammam AS, Kendzerska T, Gupta R, Ramasubramanian C, Neubauer DN, Narasimhan M, et al. Comorbid depression in obstructive sleep apnea: an under-recognized association. Sleep Breath. 2016;20(2):447-456. https://doi.org/10.1007/s11325-015-1223-x
75. Schiza SE, Bouloukaki I. Screening for obstructive sleep apnoea in professional drivers. Breathe (Sheff). 2020;16(1):29364. https://doi.org/10.1183/20734735.0364-2019
76. Strohl KP, Brown DB, Collop N, George C, Grunstein R, Han F, et al. An official American Thoracic Society Clinical Practice Guideline: sleep apnea, sleepiness, and driving risk in noncommercial drivers. An update of a 1994 Statement. Am J Respir Crit Care Med. 2013;187(11):1259-1266. https://doi.org/10.1164/rccm.201304-0726ST
77. Marrone O, Bonsignore MR. Obstructive sleep apnea and cancer: a complex relationship. Curr Opin Pulm Med. 2020;26(6):657-667. https://doi.org/10.1097/MCP.0000000000000729
78. Gozal D, Farré R, Nieto FJ. Obstructive sleep apnea and cancer: Epidemiologic links and theoretical biological constructs. Sleep Med Rev. 2016;27:43-55. https://doi.org/10.1016/j.smrv.2015.05.006
79. Cheng Y, Wang Y, Dai L. The prevalence of obstructive sleep apnea in interstitial lung disease: a systematic review and meta-analysis [published correction appears in Sleep Breath. 2021 Mar 23;:]. Sleep Breath. 2021;25(3):1219-1228. https://doi.org/10.1007/s11325-020-02282-z
80. Gille T, Didier M, Boubaya M, Moya L, Sutton A, Carton Z, et al. Obstructive sleep apnoea and related comorbidities in incident idiopathic pulmonary fibrosis. Eur Respir J. 2017;49(6):1601934. https://doi.org/10.1183/13993003.01934-2016
81. Li D, Wang B, Liu Y, Wang H. Prevalence and impact of comorbid obstructive sleep apnoea in diffuse parenchymal lung diseases. PLoS One. 2021;16(2):e0246878. https://doi.org/10.1371/journal.pone.0246878
82. Papadogiannis G, Bouloukaki I, Mermigkis C, Michelakis S, Ermidou C, Mauroudi E, et al. Patients with idiopathic pulmonary fibrosis with and without obstructive sleep apnea: differences in clinical characteristics, clinical outcomes, and the effect of PAP treatment. J Clin Sleep Med. 2021;17(3):533-544. https://doi.org/10.5664/jcsm.8932
83. Damianaki A, Vagiakis E, Sigala I, Pataka A, Rovina N, Vlachou A, et al. Τhe Co-Existence of Obstructive Sleep Apnea and Bronchial Asthma: Revelation of a New Asthma Phenotype?. J Clin Med. 2019;8(9):1476. https://doi.org/10.3390/jcm8091476
84. Wang TY, Lo YL, Lin SM, Huang CD, Chung FT, Lin HC, et al. Obstructive sleep apnoea accelerates FEV1 decline in asthmatic patients. BMC Pulm Med. 2017;17(1):55. https://doi.org/10.1186/s12890-017-0398-2
85. Kauppi P, Bachour P, Maasilta P, Bachour A. Long-term CPAP treatment improves asthma control in patients with asthma and obstructive sleep apnoea. Sleep Breath. 2016;20(4):1217-1224. https://doi.org/10.1007/s11325-016-1340-1
86. Serrano-Pariente J, Plaza V, Soriano JB, Mayos M, López-Viña A, Picado C, et al. Asthma outcomes improve with continuous positive airway pressure for obstructive sleep apnea. Allergy. 2017;72(5):802-812. https://doi.org/10.1111/all.13070
87. Davies SE, Bishopp A, Wharton S, Turner AM, Mansur AH. Does Continuous Positive Airway Pressure (CPAP) treatment of obstructive sleep apnoea (OSA) improve asthma-related clinical outcomes in patients with co-existing conditions?- A systematic review. Respir Med. 2018;143:18-30. https://doi.org/10.1016/j.rmed.2018.08.004
88. Brennan M, McDonnell MJ, Walsh SM, Gargoum F, Rutherford R. Review of the prevalence, pathogenesis and management of OSA-COPD overlap [published online ahead of print, 2022 Jan 16]. Sleep Breath. 2022;10.1007/s11325-021-02540-8. https://doi.org/10.1007/s11325-021-02540-8
89. McNicholas WT. COPD-OSA Overlap Syndrome: Evolving Evidence Regarding Epidemiology, Clinical Consequences, and Management. Chest. 2017;152(6):1318-1326. https://doi.org/10.1016/j.chest.2017.04.160
90. Adler D, Bailly S, Benmerad M, Joyeux-Faure M, Jullian-Desayes I, Soccal PM, et al. Clinical presentation and comorbidities of obstructive sleep apnea-COPD overlap syndrome. PLoS One. 2020;15(7):e0235331. https://doi.org/10.1371/journal.pone.0235331
91. Soler X, Liao SY, Marin JM, Lorenzi-Filho G, Jen R, DeYoung P, et al. Age, gender, neck circumference, and Epworth sleepiness scale do not predict obstructive sleep apnea (OSA) in moderate to severe chronic obstructive pulmonary disease (COPD): The challenge to predict OSA in advanced COPD. PLoS One. 2017;12(5):e0177289. https://doi.org/10.1371/journal.pone.0177289
92. Chaouat A, Bugnet AS, Kadaoui N, Schott R, Enache I, Ducoloné A, et al. Severe pulmonary hypertension and chronic obstructive pulmonary disease. Am J Respir Crit Care Med. 2005;172(2):189-194. https://doi.org/10.1164/rccm.200401-006OC
93. Kendzerska T, Leung RS, Aaron SD, Ayas N, Sandoz JS, Gershon AS. Cardiovascular Outcomes and All-Cause Mortality in Patients with Obstructive Sleep Apnea and Chronic Obstructive Pulmonary Disease (Overlap Syndrome). Ann Am Thorac Soc. 2019;16(1):71-81. https://doi.org/10.1513/AnnalsATS.201802-136OC
94. Marin JM, Soriano JB, Carrizo SJ, Boldova A, Celli BR. Outcomes in patients with chronic obstructive pulmonary disease and obstructive sleep apnea: the overlap syndrome. Am J Respir Crit Care Med. 2010;182(3):325-331. https://doi.org/10.1164/rccm.200912-1869OC
95. Machado MC, Vollmer WM, Togeiro SM, Bilderback AL, Oliveira MV, Leitão FS, et al. CPAP and survival in moderate-to-severe obstructive sleep apnoea syndrome and hypoxaemic COPD. Eur Respir J. 2010;35(1):132-137. https://doi.org/10.1183/09031936.00192008
96. Adir Y, Humbert M, Chaouat A. Sleep-related breathing disorders and pulmonary hypertension. Eur Respir J. 2021;57(1):2002258. https://doi.org/10.1183/13993003.02258-2020
97. Jilwan FN, Escourrou P, Garcia G, Jaïs X, Humbert M, Roisman G. High occurrence of hypoxemic sleep respiratory disorders in precapillary pulmonary hypertension and mechanisms. Chest. 2013;143(1):47-55. https://doi.org/10.1378/chest.11-3124
98. Prisco DL, Sica AL, Talwar A, Narasimhan M, Omonuwa K, Hakimisefat B, et al. Correlation of pulmonary hypertension severity with metrics of comorbid sleep-disordered breathing. Sleep Breath. 2011;15(4):633-639. https://doi.org/10.1007/s11325-010-0411-y
99. Wong HS, Williams AJ, Mok Y. The relationship between pulmonary hypertension and obstructive sleep apnea. Curr Opin Pulm Med. 2017;23(6):517-521. https://doi.org/10.1097/MCP.0000000000000421
100. Nagaoka M, Goda A, Takeuchi K, Kikuchi H, Finger M, Inami T, et al. Nocturnal Hypoxemia, But Not Sleep Apnea, Is Associated With a Poor Prognosis in Patients With Pulmonary Arterial Hypertension. Circ J. 2018;82(12):3076-3081. https://doi.org/10.1253/circj.CJ-18-0636
101. Myers KA, Mrkobrada M, Simel DL. Does this patient have obstructive sleep apnea?: The Rational Clinical Examination systematic review. JAMA. 2013;310(7):731-741. https://doi.org/10.1001/jama.2013.276185
102. Zonato AI, Martinho FL, Bittencourt LR, de Oliveira Camponês Brasil O, Gregório LC, Tufik S. Head and neck physical examination: comparison between nonapneic and obstructive sleep apnea patients. Laryngoscope. 2005;115(6):1030-1034. https://doi.org/10.1097/01.MLG.0000163494.19965.DC
103. Basoglu OK, Tasbakan MS. Gender differences in clinical and polysomnographic features of obstructive sleep apnea: a clinical study of 2827 patients. Sleep Breath. 2018;22(1):241-249. https://doi.org/10.1007/s11325-017-1482-9
104. Yu JL, Rosen I. Utility of the modified Mallampati grade and Friedman tongue position in the assessment of obstructive sleep apnea. J Clin Sleep Med. 2020;16(2):303-308. https://doi.org/10.5664/jcsm.8188
105. Esteller E, Carrasco M, Díaz-Herrera MÁ, Vila J, Sampol G, Juvanteny J, et al. Clinical Practice Guideline recommendations on examination of the upper airway for adults with suspected obstructive sleep apnoea-hypopnoea syndrome. Acta Otorrinolaringol Esp (Engl Ed). 2019;70(6):364-372. https://doi.org/10.1016/j.otorri.2018.06.008
106. Friedman M, Ibrahim H, Joseph NJ. Staging of obstructive sleep apnea/hypopnea syndrome: a guide to appropriate treatment. Laryngoscope. 2004;114(3):454-459. https://doi.org/10.1097/00005537-200403000-00013
107. Senaratna CV, Perret JL, Matheson MC, Lodge CJ, Lowe AJ, Cassim R, et al. Validity of the Berlin questionnaire in detecting obstructive sleep apnea: A systematic review and meta-analysis. Sleep Med Rev. 2017;36:116-124. https://doi.org/10.1016/j.smrv.2017.04.001
108. Jonas DE, Amick HR, Feltner C, Weber RP, Arvanitis M, Stine A, et al. Screening for Obstructive Sleep Apnea in Adults: Evidence Report and Systematic Review for the US Preventive Services Task Force [published correction appears in JAMA. 2017 Mar 28;317(12 ):1278]. JAMA. 2017;317(4):415-433. https://doi.org/10.1001/jama.2016.19635
109. Andrade L, Paiva T. Ambulatory Versus Laboratory Polysomnography in Obstructive Sleep Apnea: Comparative Assessment of Quality, Clinical Efficacy, Treatment Compliance, and Quality of Life. J Clin Sleep Med. 2018;14(8):1323-1331. https://doi.org/10.5664/jcsm.7264
110. Rosen IM, Kirsch DB, Carden KA, Malhotra RK, Ramar K, Aurora RN, et al. Clinical Use of a Home Sleep Apnea Test: An Updated American Academy of Sleep Medicine Position Statement. J Clin Sleep Med. 2018;14(12):2075-2077. https://doi.org/10.5664/jcsm.7540
111. Bernhardt L, Brady EM, Freeman SC, Polmann H, Réus JC, Flores-Mir C, et al. Diagnostic accuracy of screening questionnaires for obstructive sleep apnoea in adults in different clinical cohorts: a systematic review and meta-analysis. Sleep Breath. 2021;1-26. https://doi.org/10.1007/s11325-021-02450-9
112. Kaw R, Michota F, Jaffer A, Ghamande S, Auckley D, Golish J. Unrecognized sleep apnea in the surgical patient: implications for the perioperative setting. Chest. 2006;129(1):198-205. https://doi.org/10.1378/chest.129.1.198
113. Mutter TC, Chateau D, Moffatt M, Ramsey C, Roos LL, Kryger M. A matched cohort study of postoperative outcomes in obstructive sleep apnea: could preoperative diagnosis and treatment prevent complications?. Anesthesiology. 2014;121(4):707-718. https://doi.org/10.1097/ALN.0000000000000407
114. Abrishami A, Khajehdehi A, Chung F. A systematic review of screening questionnaires for obstructive sleep apnea. Can J Anaesth. 2010;57(5):423-438. https://doi.org/10.1007/s12630-010-9280-x
115. Ramachandran SK, Josephs LA. A meta-analysis of clinical screening tests for obstructive sleep apnea. Anesthesiology. 2009;110(4):928-939. https://doi.org/10.1097/ALN.0b013e31819c47b6
116. Netzer NC, Stoohs RA, Netzer CM, Clark K, Strohl KP. Using the Berlin Questionnaire to identify patients at risk for the sleep apnea syndrome. Ann Intern Med. 1999;131(7):485-491. https://doi.org/10.7326/0003-4819-131-7-199910050-00002
117. Chung F, Yegneswaran B, Liao P, Chung SA, Vairavanathan S, Islam S, et al. STOP questionnaire: a tool to screen patients for obstructive sleep apnea. Anesthesiology. 2008;108(5):812-821. https://doi.org/10.1097/ALN.0b013e31816d83e4
118. Marti-Soler H, Hirotsu C, Marques-Vidal P, Vollenweider P, Waeber G, Preisig M, et al. The NoSAS score for screening of sleep-disordered breathing: a derivation and validation study. Lancet Respir Med. 2016;4(9):742-748. https://doi.org/10.1016/S2213-2600(16)30075-3
119. Duarte RL, Magalhães-da-Silveira FJ, Oliveira-E-Sá TS, Silva JA, Mello FC, Gozal D. Obstructive Sleep Apnea Screening with a 4-Item Instrument, Named GOAL Questionnaire: Development, Validation and Comparative Study with No-Apnea, STOP-Bang, and NoSAS. Nat Sci Sleep. 2020;12:57-67. https://doi.org/10.2147/NSS.S238255
120. Johns MW. A new method for measuring daytime sleepiness: the Epworth sleepiness scale. Sleep. 1991;14(6):540-545. https://doi.org/10.1093/sleep/14.6.540
121. Panchasara B, Poots AJ, Davies G. Are the Epworth Sleepiness Scale and Stop-Bang model effective at predicting the severity of obstructive sleep apnoea (OSA); in particular OSA requiring treatment?. Eur Arch Otorhinolaryngol. 2017;274(12):4233-4239.
122. Grewe FA, Roeder M, Bradicich M, Schwarz EI, Held U, Thiel S, et al. Low repeatability of Epworth Sleepiness Scale after short intervals in a sleep clinic population. J Clin Sleep Med. 2020;16(5):757-764. https://doi.org/10.5664/jcsm.8350
123. Campbell AJ, Neill AM, Scott DAR. Clinical Reproducibility of the Epworth Sleepiness Scale for Patients With Suspected Sleep Apnea. J Clin Sleep Med. 2018;14(5):791-795. https://doi.org/10.5664/jcsm.7108
124. Bertolazi AN, Fagondes SC, Hoff LS, Pedro VD, Menna Barreto SS, Johns MW. Portuguese-language version of the Epworth sleepiness scale: validation for use in Brazil. J Bras Pneumol. 2009;35(9):877-883. https://doi.org/10.1590/S1806-37132009000900009
125. Andrechuk CRS, Netzer N, Zancanella E, Almeida AR, Ceolim MF. Cultural adaptation and evaluation of the measurement properties of the Berlin Questionnaire for Brazil. Sleep Med. 2019;60:182-187. https://doi.org/10.1016/j.sleep.2019.03.022
126. Ahmadi N, Chung SA, Gibbs A, Shapiro CM. The Berlin questionnaire for sleep apnea in a sleep clinic population: relationship to polysomnographic measurement of respiratory disturbance. Sleep Breath. 2008;12(1):39-45. https://doi.org/10.1007/s11325-007-0125-y
127. Nagappa M, Liao P, Wong J, Auckley D, Ramachandran SK, Memtsoudis S, et al. Validation of the STOP-Bang Questionnaire as a Screening Tool for Obstructive Sleep Apnea among Different Populations: A Systematic Review and Meta-Analysis. PLoS One. 2015;10(12):e0143697. https://doi.org/10.1371/journal.pone.0143697
128. Duarte RLM, Fonseca LBM, Magalhães-da-Silveira FJ, Silveira EAD, Rabahi MF. Validation of the STOP-Bang questionnaire as a means of screening for obstructive sleep apnea in adults in Brazil. J Bras Pneumol. 2017;43(6):456-463. https://doi.org/10.1590/s1806-37562017000000139
129. Chen H, Zheng Z, Chen R, Zeng Y, Li N, Zhu J, et al. A meta-analysis of the diagnostic value of NoSAS in patients with sleep apnea syndrome. Sleep Breath. 2022;26(2):519-531. https://doi.org/10.1007/s11325-021-02410-3
130. Duarte RLM, Magalhães-da-Silveira FJ, Gozal D. Validation of the GOAL Questionnaire as an Obstructive Sleep Apnea Screening Instrument in Bariatric Surgery Candidates: a Brazilian Single-Center Study. Obes Surg. 2020;30(12):4802-4809. https://doi.org/10.1007/s11695-020-04888-4
131. Duarte RLM, Magalhães-da-Silveira FJ, Gozal D. Prediction of obstructive sleep apnea using GOAL questionnaire in adults with or without excessive daytime sleepiness: A cross-sectional study. Sleep Health. 2021;7(2):212-218. https://doi.org/10.1016/j.sleh.2021.01.003
132. Kushida CA, Littner MR, Morgenthaler T, Alessi CA, Bailey D, Coleman J Jr, et al. Practice parameters for the indications for polysomnography and related procedures: an update for 2005. Sleep. 2005;28(4):499-521. https://doi.org/10.1093/sleep/28.4.499
133. Berry RB, Budhiraja R, Gottlieb DJ, Gozal D, Iber C, Kapur VK, et al. Rules for scoring respiratory events in sleep: update of the 2007 AASM Manual for the Scoring of Sleep and Associated Events. Deliberations of the Sleep Apnea Definitions Task Force of the American Academy of Sleep Medicine. J Clin Sleep Med. 2012;8(5):597-619. https://doi.org/10.5664/jcsm.2172
134. Chediak AD, Acevedo-Crespo JC, Seiden DJ, Kim HH, Kiel MH. Nightly variability in the indices of sleep-disordered breathing in men being evaluated for impotence with consecutive night polysomnograms. Sleep. 1996;19(7):589-592. https://doi.org/10.1093/sleep/19.7.589
135. Roeder M, Bradicich M, Schwarz EI, Thiel S, Gaisl T, Held U, et al. Night-to-night variability of respiratory events in obstructive sleep apnoea: a systematic review and meta-analysis. Thorax. 2020;75(12):1095-1102. https://doi.org/10.1136/thoraxjnl-2020-214544
136. Duce B, Milosavljevic J, Hukins C. The 2012 AASM Respiratory Event Criteria Increase the Incidence of Hypopneas in an Adult Sleep Center Population. J Clin Sleep Med. 2015;11(12):1425-1431. https://doi.org/10.5664/jcsm.5280
137. Ho V, Crainiceanu CM, Punjabi NM, Redline S, Gottlieb DJ. Calibration Model for Apnea-Hypopnea Indices: Impact of Alternative Criteria for Hypopneas. Sleep. 2015;38(12):1887-1892. https://doi.org/10.5665/sleep.5234
138. Khan Y, Heckmatt JZ. Obstructive apnoeas in Duchenne muscular dystrophy. Thorax. 1994;49(2):157-161. https://doi.org/10.1136/thx.49.2.157
139. Rodrigues Filho JC, Neves DD, Moreira GA, Viana ADC Jr, Araújo-Melo MH. Nocturnal oximetry in the diagnosis of obstructive sleep apnea syndrome in potentially hypoxic patients due to neuromuscular diseases. Sleep Med. 2021;84:127-133. https://doi.org/10.1016/j.sleep.2021.05.009
140. Collop NA, Anderson WM, Boehlecke B, Claman D, Goldberg R, Gottlieb DJ, et al. Clinical guidelines for the use of unattended portable monitors in the diagnosis of obstructive sleep apnea in adult patients. Portable Monitoring Task Force of the American Academy of Sleep Medicine. J Clin Sleep Med. 2007;3(7):737-747. https://doi.org/10.5664/jcsm.27032
141. Nigro CA, Dibur E, Aimaretti S, González S, Rhodius E. Comparison of the automatic analysis versus the manual scoring from ApneaLink™ device for the diagnosis of obstructive sleep apnoea syndrome. Sleep Breath. 2011;15(4):679-686. https://doi.org/10.1007/s11325-010-0421-9
142. Labarca G, Dreyse J, Salas C, Contreras A, Nazar G, Gaete MI, et al. Differences between manual and automatic analysis in determining the severity of obstructive sleep apnea using home sleep apnea testing. Sleep Med. 2018;47:66-71. https://doi.org/10.1016/j.sleep.2018.03.015
143. Zancanella E, do Prado LF, de Carvalho LB, Machado Júnior AJ, Crespo AN, do Prado GF. Home sleep apnea testing: an accuracy study. Sleep Breath. 2022;26(1):117-123. https://doi.org/10.1007/s11325-021-02372-6
144. Caples SM, Anderson WM, Calero K, Howell M, Hashmi SD. Use of polysomnography and home sleep apnea tests for the longitudinal management of obstructive sleep apnea in adults: an American Academy of Sleep Medicine clinical guidance statement. J Clin Sleep Med. 2021;17(6):1287-1293. https://doi.org/10.5664/jcsm.9240
145. Behar JA, Palmius N, Zacharie S, Chocron A, Penzel T, Bittencourt L, et al. ingle-channel oximetry monitor versus in-lab polysomnography oximetry analysis: does it make a difference?. Physiol Meas. 2020;41(4):044007. https://doi.org/10.1088/1361-6579/ab8856
146. Pinheiro GDL, Cruz AF, Domingues DM, Genta PR, Drager LF, Strollo PJ, et al. Validation of an Overnight Wireless High-Resolution Oximeter plus Cloud-Based Algorithm for the Diagnosis of Obstructive Sleep Apnea. Clinics (Sao Paulo). 2020;75:e2414. https://doi.org/10.6061/clinics/2020/e2414
147. Rodrigues Filho JC, Neves DD, Velasque L, Maranhão AA, de Araujo-Melo MH. Diagnostic performance of nocturnal oximetry in the detection of obstructive sleep apnea syndrome: a Brazilian study. Sleep Breath. 2020;24(4):1487-1494. https://doi.org/10.1007/s11325-019-02000-4
148. Del Campo F, Crespo A, Cerezo-Hernández A, Gutiérrez-Tobal GC, Hornero R, Álvarez D. Oximetry use in obstructive sleep apnea. Expert Rev Respir Med. 2018;12(8):665-681. https://doi.org/10.1080/17476348.2018.1495563
149. Andrés-Blanco AM, Álvarez D, Crespo A, Arroyo CA, Cerezo-Hernández A, Gutiérrez-Tobal GC, et al. Assessment of automated analysis of portable oximetry as a screening test for moderate-to-severe sleep apnea in patients with chronic obstructive pulmonary disease. PLoS One. 2017;12(11):e0188094. https://doi.org/10.1371/journal.pone.0188094
150. Patil SP, Ayappa IA, Caples SM, Kimoff RJ, Patel SR, Harrod CG. Treatment of Adult Obstructive Sleep Apnea with Positive Airway Pressure: An American Academy of Sleep Medicine Clinical Practice Guideline. J Clin Sleep Med. 2019;15(2):335-343. https://doi.org/10.5664/jcsm.7640
151. Patil SP, Ayappa IA, Caples SM, Kimoff RJ, Patel SR, Harrod CG. Treatment of Adult Obstructive Sleep Apnea With Positive Airway Pressure: An American Academy of Sleep Medicine Systematic Review, Meta-Analysis, and GRADE Assessment. J Clin Sleep Med. 2019;15(2):301-334. https://doi.org/10.5664/jcsm.7638
152. Clinical guidelines for the manual titration of positive airway pressure in patients with obstructive sleep apnea. J Clin Sleep Med. 2008;4(2):157-171. https://doi.org/10.5664/jcsm.27133
153. Goh KJ, Soh RY, Leow LC, Toh ST, Song PR, Hao Y, et al. Choosing the right mask for your Asian patient with sleep apnoea: A randomized, crossover trial of CPAP interfaces. Respirology. 2019;24(3):278-285. https://doi.org/10.1111/resp.13396
154. Zonato AI, Rosa CFA, Oliveira L, Bittencourt LR. Efficacy of nasal masks versus nasal pillows masks during continuous positive airway pressure titration for patients with obstructive sleep apnea. Sleep Breath. 2021;25(3):1-8. https://doi.org/10.1007/s11325-020-02251-6
155. Duarte RLM, Mendes BA, Oliveira-E-Sá TS, Magalhães-da-Silveira FJ, Gozal D. Nasal versus oronasal mask in patients under auto-adjusting continuous positive airway pressure titration: a real-life study. Eur Arch Otorhinolaryngol. 2020;277(12):3507-3512. https://doi.org/10.1007/s00405-020-06242-x
156. Genta PR, Kaminska M, Edwards BA, Ebben MR, Krieger AC, Tamisier R, et al. The Importance of Mask Selection on Continuous Positive Airway Pressure Outcomes for Obstructive Sleep Apnea. An Official American Thoracic Society Workshop Report. Ann Am Thorac Soc. 2020;17(10):1177-1185. https://doi.org/10.1513/AnnalsATS.202007-864ST
157. Cassel W, Canisius S, Becker HF, Leistner S, Ploch T, Jerrentrup A, et al. A prospective polysomnographic study on the evolution of complex sleep apnoea. Eur Respir J. 2011;38(2):329-337. https://doi.org/10.1183/09031936.00162009
158. Zeineddine S, Badr MS. Treatment-Emergent Central Apnea: Physiologic Mechanisms Informing Clinical Practice. Chest. 2021;159(6):2449-2457. https://doi.org/10.1016/j.chest.2021.01.036
159. Khan MT, Franco RA. Complex sleep apnea syndrome. Sleep Disord. 2014;2014:798487. https://doi.org/10.1155/2014/798487
160. Veasey SC, Rosen IM. Obstructive Sleep Apnea in Adults. N Engl J Med. 2019;380(15):1442-1449. https://doi.org/10.1056/NEJMcp1816152
161. Masa JF, Jiménez A, Durán J, Capote F, Monasterio C, Mayos M, et al. Alternative methods of titrating continuous positive airway pressure: a large multicenter study. Am J Respir Crit Care Med. 2004;170(11):1218-1224. https://doi.org/10.1164/rccm.200312-1787OC
162. Kim H, Lee M, Hwangbo Y, Yang KI. Automatic Derivation of Continuous Positive Airway Pressure Settings: Comparison with In-Laboratory Titration. J Clin Neurol. 2020;16(2):314-320. https://doi.org/10.3988/jcn.2020.16.2.314
163. Gao W, Jin Y, Wang Y, Sun M, Chen B, Zhou N, et al. Is automatic CPAP titration as effective as manual CPAP titration in OSAHS patients? A meta-analysis. Sleep Breath. 2012;16(2):329-340. https://doi.org/10.1007/s11325-011-0495-z
164. Mulgrew AT, Fox N, Ayas NT, Ryan CF. Diagnosis and initial management of obstructive sleep apnea without polysomnography: a randomized validation study. Ann Intern Med. 2007;146(3):157-166. https://doi.org/10.7326/0003-4819-146-3-200702060-00004
165. Rotenberg BW, Murariu D, Pang KP. Trends in CPAP adherence over twenty years of data collection: a flattened curve. J Otolaryngol Head Neck Surg. 2016;45(1):43. https://doi.org/10.1186/s40463-016-0156-0
166. Mashaqi S, Gozal D. The impact of obstructive sleep apnea and PAP therapy on all-cause and cardiovascular mortality based on age and gender - a literature review. Respir Investig. 2020;58(1):7-20. https://doi.org/10.1016/j.resinv.2019.08.002
167. Weaver TE, Maislin G, Dinges DF, Bloxham T, George CF, Greenberg H, et al. Relationship between hours of CPAP use and achieving normal levels of sleepiness and daily functioning. Sleep. 2007;30(6):711-719. https://doi.org/10.1093/sleep/30.6.711
168. Martínez-García MA, Capote F, Campos-Rodríguez F, Lloberes P, Díaz de Atauri MJ, Somoza M, et al. Effect of CPAP on blood pressure in patients with obstructive sleep apnea and resistant hypertension: the HIPARCO randomized clinical trial. JAMA. 2013;310(22):2407-2415. https://doi.org/10.1001/jama.2013.281250
169. Lewis KE, Seale L, Bartle IE, Watkins AJ, Ebden P. Early predictors of CPAP use for the treatment of obstructive sleep apnea. Sleep. 2004;27(1):134-138. https://doi.org/10.1093/sleep/27.1.134
170. Pépin JL, Woehrle H, Liu D, Shao S, Armitstead JP, Cistulli PA, et al. Adherence to Positive Airway Therapy After Switching From CPAP to ASV: A Big Data Analysis. J Clin Sleep Med. 2018;14(1):57-63. https://doi.org/10.5664/jcsm.6880
171. Cistulli PA, Armitstead J, Pepin JL, Woehrle H, Nunez CM, Benjafield A, et al. Short-term CPAP adherence in obstructive sleep apnea: a big data analysis using real world data. Sleep Med. 2019;59:114-116. https://doi.org/10.1016/j.sleep.2019.01.004
172. Jacobsen AR, Eriksen F, Hansen RW, Erlandsen M, Thorup L, Damgård MB, et al. Determinants for adherence to continuous positive airway pressure therapy in obstructive sleep apnea. PLoS One. 2017;12(12):e0189614. https://doi.org/10.1371/journal.pone.0189614
173. Kohler M, Smith D, Tippett V, Stradling JR. Predictors of long-term compliance with continuous positive airway pressure. Thorax. 2010;65(9):829-832. https://doi.org/10.1136/thx.2010.135848
174. Genzor S, Prasko J, Vanek J, Asswad AG, Nadjarpour S, Sova M. Adherence of obstructive sleep apnoea syndrome patients to positive airway pressure therapy - 10-year follow-up [published online ahead of print, 2021 Jul 2]. Biomed Pap Med Fac Univ Palacky Olomouc Czech Repub. 2021;10.5507/bp.2021.041. https://doi.org/10.5507/bp.2021.041
175. Richards D, Bartlett DJ, Wong K, Malouff J, Grunstein RR. Increased adherence to CPAP with a group cognitive behavioral treatment intervention: a randomized trial. Sleep. 2007;30(5):635-640. https://doi.org/10.1093/sleep/30.5.635
176. Lettieri CJ, Shah AA, Holley AB, Kelly WF, Chang AS, Roop SA, et al. Effects of a short course of eszopiclone on continuous positive airway pressure adherence: a randomized trial. Ann Intern Med. 2009;151(10):696-702. https://doi.org/10.7326/0003-4819-151-10-200911170-00006
177. Siccoli MM, Pepperell JC, Kohler M, Craig SE, Davies RJ, Stradling JR. Effects of continuous positive airway pressure on quality of life in patients with moderate to severe obstructive sleep apnea: data from a randomized controlled trial. Sleep. 2008;31(11):1551-1558. https://doi.org/10.1093/sleep/31.11.1551
178. Tomfohr LM, Ancoli-Israel S, Loredo JS, Dimsdale JE. Effects of continuous positive airway pressure on fatigue and sleepiness in patients with obstructive sleep apnea: data from a randomized controlled trial. Sleep. 2011;34(1):121-126. https://doi.org/10.1093/sleep/34.1.121
179. George CF. Reduction in motor vehicle collisions following treatment of sleep apnoea with nasal CPAP. Thorax. 2001;56(7):508-512. https://doi.org/10.1136/thx.56.7.508
180. Faccenda JF, Mackay TW, Boon NA, Douglas NJ. Randomized placebo-controlled trial of continuous positive airway pressure on blood pressure in the sleep apnea-hypopnea syndrome. Am J Respir Crit Care Med. 2001;163(2):344-348. https://doi.org/10.1164/ajrccm.163.2.2005037
181. Myhill PC, Davis WA, Peters KE, Chubb SA, Hillman D, Davis TM. Effect of continuous positive airway pressure therapy on cardiovascular risk factors in patients with type 2 diabetes and obstructive sleep apnea. J Clin Endocrinol Metab. 2012;97(11):4212-4218. https://doi.org/10.1210/jc.2012-2107
182. Barbé F, Durán-Cantolla J, Sánchez-de-la-Torre M, Martínez-Alonso M, Carmona C, Barceló A, et al. Effect of continuous positive airway pressure on the incidence of hypertension and cardiovascular events in nonsleepy patients with obstructive sleep apnea: a randomized controlled trial. JAMA. 2012;307(20):2161-2168. https://doi.org/10.1001/jama.2012.4366
183. McEvoy RD, Antic NA, Heeley E, Luo Y, Ou Q, Zhang X, et al. Prevention of Cardiovascular Events in Obstructive Sleep Apnea. N Engl J Med. 2016;375(10):919-931. https://doi.org/10.1056/NEJMoa1606599
184. Xie J, Sert Kuniyoshi FH, Covassin N, Singh P, Gami AS, Chahal CAA, et al. Excessive Daytime Sleepiness Independently Predicts Increased Cardiovascular Risk After Myocardial Infarction. J Am Heart Assoc. 2018;7(2):e007221. https://doi.org/10.1161/JAHA.117.007221
185. Sawyer AM, Gooneratne NS, Marcus CL, Ofer D, Richards KC, Weaver TE. A systematic review of CPAP adherence across age groups: clinical and empiric insights for developing CPAP adherence interventions. Sleep Med Rev. 2011;15(6):343-356. https://doi.org/10.1016/j.smrv.2011.01.003
186. Weaver TE, Grunstein RR. Adherence to continuous positive airway pressure therapy: the challenge to effective treatment. Proc Am Thorac Soc. 2008;5(2):173-178. https://doi.org/10.1513/pats.200708-119MG
187. Shamim-Uzzaman QA, Bae CJ, Ehsan Z, Setty AR, Devine M, Dhankikar S, et al. The use of telemedicine for the diagnosis and treatment of sleep disorders: an American Academy of Sleep Medicine update. J Clin Sleep Med. 2021;17(5):1103-1107. https://doi.org/10.5664/jcsm.9194
188. Bruyneel M. Telemedicine in the diagnosis and treatment of sleep apnoea. Eur Respir Rev. 2019;28(151):180093. https://doi.org/10.1183/16000617.0093-2018
189. Schutte-Rodin S. Telehealth, Telemedicine, and Obstructive Sleep Apnea. Sleep Med Clin. 2020;15(3):359-375. https://doi.org/10.1016/j.jsmc.2020.05.003
190. Pépin JL, Tamisier R, Hwang D, Mereddy S, Parthasarathy S. Does remote monitoring change OSA management and CPAP adherence?. Respirology. 2017;22(8):1508-1517. https://doi.org/10.1111/resp.13183
191. Hoet F, Libert W, Sanida C, Van den Broecke S, Bruyneel AV, Bruyneel M. Telemonitoring in continuous positive airway pressure-treated patients improves delay to first intervention and early compliance: a randomized trial. Sleep Med. 2017;39:77-83. https://doi.org/10.1016/j.sleep.2017.08.016
192. Budhiraja R, Parthasarathy S, Drake CL, Roth T, Sharief I, Budhiraja P, et al. Early CPAP use identifies subsequent adherence to CPAP therapy. Sleep. 2007;30(3):320-324. PMID: 17425228
193. Bros JS, Poulet C, Arnol N, Deschaux C, Gandit M, Charavel M. Acceptance of Telemonitoring Among Patients with Obstructive Sleep Apnea Syndrome: How is the Perceived Interest by and for Patients?. Telemed J E Health. 2018;24(5):351-359. https://doi.org/10.1089/tmj.2017.0134
194. Chen C, Wang J, Pang L, Wang Y, Ma G, Liao W. Telemonitor care helps CPAP compliance in patients with obstructive sleep apnea: a systemic review and meta-analysis of randomized controlled trials. Ther Adv Chronic Dis. 2020;11:2040622320901625. https://doi.org/10.1177/2040622320901625
195. Labarca G, Schmidt A, Dreyse J, Jorquera J, Barbe F. Telemedicine interventions for CPAP adherence in obstructive sleep apnea patients: Systematic review and meta-analysis. Sleep Med Rev. 2021;60:101543. https://doi.org/10.1016/j.smrv.2021.101543
196. Drager LF, Malhotra A, Yan Y, Pépin JL, Armitstead JP, Woehrle H, et al. Adherence with positive airway pressure therapy for obstructive sleep apnea in developing vs. developed countries: a big data study. J Clin Sleep Med. 2021;17(4):703-709. https://doi.org/10.5664/jcsm.9008
197. Srijithesh PR, Aghoram R, Goel A, Dhanya J. Positional therapy for obstructive sleep apnoea. Cochrane Database Syst Rev. 2019;5(5):CD010990. https://doi.org/10.1002/14651858.CD010990.pub2
198. Hudgel DW, Patel SR, Ahasic AM, Bartlett SJ, Bessesen DH, Coaker MA, et al. The Role of Weight Management in the Treatment of Adult Obstructive Sleep Apnea. An Official American Thoracic Society Clinical Practice Guideline. Am J Respir Crit Care Med. 2018;198(6):e70-e87. https://doi.org/10.1164/rccm.201807-1326ST
199. Peppard PE, Young T. Exercise and sleep-disordered breathing: an association independent of body habitus. Sleep. 2004;27(3):480-484. https://doi.org/10.1093/sleep/27.3.480
200. Ramar K, Dort LC, Katz SG, Lettieri CJ, Harrod CG, Thomas SM, et al. Clinical Practice Guideline for the Treatment of Obstructive Sleep Apnea and Snoring with Oral Appliance Therapy: An Update for 2015. J Clin Sleep Med. 2015;11(7):773-827. https://doi.org/10.5664/jcsm.4858
201. Lim J, Lasserson TJ, Fleetham J, Wright J. Oral appliances for obstructive sleep apnoea. Cochrane Database Syst Rev. 2006;2006(1):CD004435. https://doi.org/10.1002/14651858.CD004435.pub3
202. Phillips CL, Grunstein RR, Darendeliler MA, Mihailidou AS, Srinivasan VK, Yee BJ, et al. Health outcomes of continuous positive airway pressure versus oral appliance treatment for obstructive sleep apnea: a randomized controlled trial. Am J Respir Crit Care Med. 2013;187(8):879-887. https://doi.org/10.1164/rccm.201212-2223OC
203. Hoffstein V. Review of oral appliances for treatment of sleep-disordered breathing. Sleep Breath. 2007;11(1):1-22. https://doi.org/10.1007/s11325-006-0084-8
204. Brimioulle M, Chaidas K. Nasal function and CPAP use in patients with obstructive sleep apnoea: a systematic review. Sleep Breath. 2021;10.1007/s11325-021-02478-x. https://doi.org/10.1007/s11325-021-02478-x
205. Aurora RN, Casey KR, Kristo D, Auerbach S, Bista SR, Chowdhuri S, et al. Practice parameters for the surgical modifications of the upper airway for obstructive sleep apnea in adults. Sleep. 2010;33(10):1408-1413. https://doi.org/10.1093/sleep/33.10.1408
206. Zaghi S, Holty JE, Certal V, Abdullatif J, Guilleminault C, Powell NB, et al. Maxillomandibular Advancement for Treatment of Obstructive Sleep Apnea: A Meta-analysis. JAMA Otolaryngol Head Neck Surg. 2016;142(1):58-66. https://doi.org/10.1001/jamaoto.2015.2678
207. Strollo PJ Jr, Soose RJ, Maurer JT, de Vries N, Cornelius J, Froymovich O, et al. Upper-airway stimulation for obstructive sleep apnea. N Engl J Med. 2014;370(2):139-149. https://doi.org/10.1056/NEJMoa1308659
208. Costantino A, Rinaldi V, Moffa A, Luccarelli V, Bressi F, Cassano M, et al. Hypoglossal nerve stimulation long-term clinical outcomes: a systematic review and meta-analysis. Sleep Breath. 2020;24(2):399-411. https://doi.org/10.1007/s11325-019-01923-2
209. Fritscher LG, Canani S, Mottin CC, Fritscher CC, Berleze D, Chapman K, et al. Bariatric surgery in the treatment of obstructive sleep apnea in morbidly obese patients. Respiration. 2007;74(6):647-652. https://doi.org/10.1159/000107736
210. Zhang Y, Wang W, Yang C, Shen J, Shi M, Wang B. Improvement in Nocturnal Hypoxemia in Obese Patients with Obstructive Sleep Apnea after Bariatric Surgery: a Meta-Analysis. Obes Surg. 2019;29(2):601-608. https://doi.org/10.1007/s11695-018-3573-5
211. Sjöström L, Narbro K, Sjöström CD, Karason K, Larsson B, Wedel H, et al. Effects of bariatric surgery on mortality in Swedish obese subjects. N Engl J Med. 2007;357(8):741-752. https://doi.org/10.1056/NEJMoa066254
212. Kong WT, Chopra S, Kopf M, Morales C, Khan S, Zuccala K, et al. Perioperative Risks of Untreated Obstructive Sleep Apnea in the Bariatric Surgery Patient: a Retrospective Study. Obes Surg. 2016;26(12):2886-2890. https://doi.org/10.1007/s11695-016-2203-3
213. Carron M, Zarantonello F, Tellaroli P, Ori C. Perioperative noninvasive ventilation in obese patients: a qualitative review and meta-analysis. Surg Obes Relat Dis. 2016;12(3):681-691. https://doi.org/10.1016/j.soard.2015.12.013
214. Buchwald H, Avidor Y, Braunwald E, Jensen MD, Pories W, Fahrbach K, et al. Bariatric surgery: a systematic review and meta-analysis [published correction appears in JAMA. 2005 Apr 13;293(14):1728]. JAMA. 2004;292(14):1724-1737. https://doi.org/10.1001/jama.292.14.1724
215. Furlan SF, Drager LF, Santos RN, Damiani LP, Bersch-Ferreira AC, Miranda TA, et al. Three-year effects of bariatric surgery on obstructive sleep apnea in patients with obesity grade 1 and 2: a sub-analysis of the GATEWAY trial. Int J Obes (Lond). 2021;45(4):914-917. https://doi.org/10.1038/s41366-021-00752-2
216. Guimarães KC, Drager LF, Genta PR, Marcondes BF, Lorenzi-Filho G. Effects of oropharyngeal exercises on patients with moderate obstructive sleep apnea syndrome. Am J Respir Crit Care Med. 2009;179(10):962-966. https://doi.org/10.1164/rccm.200806-981OC
217. Hsu B, Emperumal CP, Grbach VX, Padilla M, Enciso R. Effects of respiratory muscle therapy on obstructive sleep apnea: a systematic review and meta-analysis. J Clin Sleep Med. 2020;16(5):785-801. https://doi.org/10.5664/jcsm.8318
218. Osman AM, Carter SG, Carberry JC, Eckert DJ. Obstructive sleep apnea: current perspectives. Nat Sci Sleep. 2018;10:21-34. https://doi.org/10.2147/NSS.S124657
219. Chebbo A, Tfaili A, Jones SF. Hypoventilation syndromes. Med Clin North Am. 2011;95(6):1189-1202. https://doi.org/10.1016/j.mcna.2011.09.002
220. Brown LK. Hypoventilation syndromes. Clin Chest Med. 2010;31(2):249-270. https://doi.org/10.1016/j.ccm.2010.03.002
221. Mokhlesi B, Tulaimat A, Faibussowitsch I, Wang Y, Evans AT. Obesity hypoventilation syndrome: prevalence and predictors in patients with obstructive sleep apnea. Sleep Breath. 2007;11(2):117-124. https://doi.org/10.1007/s11325-006-0092-8
222. Mokhlesi B, Masa JF, Brozek JL, Gurubhagavatula I, Murphy PB, Piper AJ, et al. Evaluation and Management of Obesity Hypoventilation Syndrome. An Official American Thoracic Society Clinical Practice Guideline [published correction appears in Am J Respir Crit Care Med. 2019 Nov 15;200(10):1326]. Am J Respir Crit Care Med. 2019;200(3):e6-e24. https://doi.org/10.1164/rccm.201905-1071ST
223. Athayde RAB, Oliveira Filho JRB, Lorenzi Filho G, Genta PR. Obesity hypoventilation syndrome: a current review. J Bras Pneumol. 2018;44(6):510-518. https://doi.org/10.1590/s1806-37562017000000332
224. Piper AJ, Grunstein RR. Obesity hypoventilation syndrome: mechanisms and management. Am J Respir Crit Care Med. 2011;183(3):292-298. https://doi.org/10.1164/rccm.201008-1280CI
225. Benditt JO, Boitano LJ. Pulmonary issues in patients with chronic neuromuscular disease. Am J Respir Crit Care Med. 2013;187(10):1046-1055. https://doi.org/10.1164/rccm.201210-1804CI
226. Baydur A, Layne E, Aral H, Krishnareddy N, Topacio R, Frederick G, et al. Long term non-invasive ventilation in the community for patients with musculoskeletal disorders: 46 year experience and review. Thorax. 2000;55(1):4-11. https://doi.org/10.1136/thorax.55.1.4
227. Buyse B, Meersseman W, Demedts M. Treatment of chronic respiratory failure in kyphoscoliosis: oxygen or ventilation?. Eur Respir J. 2003;22(3):525-528. https://doi.org/10.1183/09031936.03.00076103
228. Vanderlaan M, Holbrook CR, Wang M, Tuell A, Gozal D. Epidemiologic survey of 196 patients with congenital central hypoventilation syndrome. Pediatr Pulmonol. 2004;37(3):217-229. https://doi.org/10.1002/ppul.10438
229. Windisch W, Hennings E, Storre JH, Matthys H, Sorichter S. Long-term survival of a patient with congenital central hypoventilation syndrome despite the lack of continuous ventilatory support. Respiration. 2004;71(2):195-198. https://doi.org/10.1159/000076685
230. Weese-Mayer DE, Silvestri JM, Kenny AS, Ilbawi MN, Hauptman SA, Lipton JW, et al. Diaphragm pacing with a quadripolar phrenic nerve electrode: an international study. Pacing Clin Electrophysiol. 1996;19(9):1311-1319. https://doi.org/10.1111/j.1540-8159.1996.tb04209.x
231. Weitzenblum E, Chaouat A, Kessler R, Canuet M. Overlap syndrome: obstructive sleep apnea in patients with chronic obstructive pulmonary disease. Proc Am Thorac Soc. 2008;5(2):237-241. https://doi.org/10.1513/pats.200706-077MG
232. Owens RL, Malhotra A. Sleep-disordered breathing and COPD: the overlap syndrome. Respir Care. 2010;55(10):1333-1346.
233. Muza RT. Central sleep apnoea-a clinical review. J Thorac Dis. 2015;7(5):930-937. https://doi.org/10.3978/j.issn.2072-1439.2015.04.45
234. Ishikawa O, Oks M. Central Sleep Apnea. Clin Geriatr Med. 2021;37(3):469-481. https://doi.org/10.1016/j.cger.2021.04.009
235. Eckert DJ, Jordan AS, Merchia P, Malhotra A. Central sleep apnea: Pathophysiology and treatment. Chest. 2007;131(2):595-607. https://doi.org/10.1378/chest.06.2287
236. White DP. Pathogenesis of obstructive and central sleep apnea. Am J Respir Crit Care Med. 2005;172(11):1363-1370. https://doi.org/10.1164/rccm.200412-1631SO
237. Donovan LM, Kapur VK. Prevalence and Characteristics of Central Compared to Obstructive Sleep Apnea: Analyses from the Sleep Heart Health Study Cohort. Sleep. 2016;39(7):1353-1359. https://doi.org/10.5665/sleep.5962
238. Sin DD, Fitzgerald F, Parker JD, Newton G, Floras JS, Bradley TD. Risk factors for central and obstructive sleep apnea in 450 men and women with congestive heart failure. Am J Respir Crit Care Med. 1999;160(4):1101-1106. https://doi.org/10.1164/ajrccm.160.4.9903020
239. Oldenburg O, Lamp B, Faber L, Teschler H, Horstkotte D, Töpfer V. Sleep-disordered breathing in patients with symptomatic heart failure: a contemporary study of prevalence in and characteristics of 700 patients. Eur J Heart Fail. 2007;9(3):251-257. https://doi.org/10.1016/j.ejheart.2006.08.003
240. Küpper T, Schöffl V, Netzer N. Cheyne stokes breathing at high altitude: a helpful response or a troublemaker?. Sleep Breath. 2008;12(2):123-127. https://doi.org/10.1007/s11325-007-0155-5
241. Schütz SG, Lisabeth LD, Hsu CW, Kim S, Chervin RD, Brown DL. Central sleep apnea is uncommon after stroke. Sleep Med. 2021;77:304-306. https://doi.org/10.1016/j.sleep.2020.08.025
242. Seiler A, Camilo M, Korostovtseva L, Haynes AG, Brill AK, Horvath T, et al. Prevalence of sleep-disordered breathing after stroke and TIA: A meta-analysis. Neurology. 2019;92(7):e648-e654. https://doi.org/10.1212/WNL.0000000000006904
243. Pattinson KT. Opioids and the control of respiration. Br J Anaesth. 2008;100(6):747-758. https://doi.org/10.1093/bja/aen094
244. Wang D, Teichtahl H, Drummer O, Goodman C, Cherry G, Cunnington D, et al. Central sleep apnea in stable methadone maintenance treatment patients. Chest. 2005;128(3):1348-1356. https://doi.org/10.1378/chest.128.3.1348
245. Randerath W, Verbraecken J, Andreas S, Arzt M, Bloch KE, Brack T, et al. Definition, discrimination, diagnosis and treatment of central breathing disturbances during sleep. Eur Respir J. 2017;49(1):1600959. https://doi.org/10.1183/13993003.00959-2016
246. Bradley TD, Logan AG, Kimoff RJ, Sériès F, Morrison D, Ferguson K, et al. Continuous positive airway pressure for central sleep apnea and heart failure. N Engl J Med. 2005;353(19):2025-2033. https://doi.org/10.1056/NEJMoa051001
247. Granton JT, Naughton MT, Benard DC, Liu PP, Goldstein RS, Bradley TD. CPAP improves inspiratory muscle strength in patients with heart failure and central sleep apnea. Am J Respir Crit Care Med. 1996;153(1):277-282. https://doi.org/10.1164/ajrccm.153.1.8542129
248. Cowie MR, Woehrle H, Wegscheider K, Angermann C, d’Ortho MP, Erdmann E, et al. Adaptive Servo-Ventilation for Central Sleep Apnea in Systolic Heart Failure. N Engl J Med. 2015;373(12):1095-1105. https://doi.org/10.1056/NEJMoa1506459
249. Potratz M, Sohns C, Dumitrescu D, Sommer P, Fox H. Phrenic Nerve Stimulation Improves Physical Performance and Hypoxemia in Heart Failure Patients with Central Sleep Apnea. J Clin Med. 2021;10(2):202. https://doi.org/10.3390/jcm10020202
250. Kaditis AG, Alonso Alvarez ML, Boudewyns A, Alexopoulos EI, Ersu R, Joosten K, et al. Obstructive sleep disordered breathing in 2- to 18-year-old children: diagnosis and management. Eur Respir J. 2016;47(1):69-94. https://doi.org/10.1183/13993003.00385-2015
251. Lumeng JC, Chervin RD. Epidemiology of pediatric obstructive sleep apnea. Proc Am Thorac Soc. 2008;5(2):242-252. https://doi.org/10.1513/pats.200708-135MG
252. Gipson K, Lu M, Kinane TB. Sleep-Disordered Breathing in Children [published correction appears in Pediatr Rev. 2019 May;40(5):261]. Pediatr Rev. 2019;40(1):3-13. https://doi.org/10.1542/pir.2018-0142
253. Schwengel DA, Dalesio NM, Stierer TL. Pediatric obstructive sleep apnea. Anesthesiol Clin. 2014;32(1):237-261. https://doi.org/10.1016/j.anclin.2013.10.012
254. Marcus CL, Brooks LJ, Draper KA, Gozal D, Halbower AC, Jones J, et al. Diagnosis and management of childhood obstructive sleep apnea syndrome. Pediatrics. 2012;130(3):576-584. https://doi.org/10.1542/peds.2012-1671
255. Sleep-related breathing disorders in adults: recommendations for syndrome definition and measurement techniques in clinical research. The Report of an American Academy of Sleep Medicine Task Force. Sleep. 1999;22(5):667-689. https://doi.org/10.1093/sleep/22.5.667