ABSTRACT
Chronic unexplained dyspnea and exercise intolerance represent common, distressing symptoms in outpatients. Clinical history taking and physical examination are the mainstays for diagnostic evaluation. However, the cause of dyspnea may remain elusive even after comprehensive diagnostic evaluation-basic laboratory analyses; chest imaging; pulmonary function testing; and cardiac testing. At that point (and frequently before), patients are usually referred to a pulmonologist, who is expected to be the main physician to solve this conundrum. In this context, cardiopulmonary exercise testing (CPET), to assess physiological and sensory responses from rest to peak exercise, provides a unique opportunity to unmask the mechanisms of the underlying dyspnea and their interactions with a broad spectrum of disorders. However, CPET is underused in clinical practice, possibly due to operational issues (equipment costs, limited availability, and poor remuneration) and limited medical education regarding the method. To counter the latter shortcoming, we aspire to provide a pragmatic strategy for interpreting CPET results. Clustering findings of exercise response allows the characterization of patterns that permit the clinician to narrow the list of possible diagnoses rather than pinpointing a specific etiology. We present a proposal for a diagnostic workup and some illustrative cases assessed by CPET. Given that airway hyperresponsiveness and pulmonary vascular disorders, which are within the purview of pulmonology, are common causes of chronic unexplained dyspnea, we also aim to describe the role of bronchial challenge tests and the diagnostic reasoning for investigating the pulmonary circulation in this context.
Keywords:
Dyspnea; Exercise test; Bronchial provocation tests; Vascular diseases.
RESUMO
A dispneia crônica inexplicada e a intolerância ao exercício representam sintomas comuns e angustiantes em pacientes ambulatoriais. O histórico clínico e o exame físico são as bases da avaliação diagnóstica. No entanto, a causa da dispneia pode permanecer inexplicada mesmo após uma avaliação diagnóstica abrangente - análises laboratoriais básicas, exames de imagem do tórax, testes de função pulmonar e testes cardíacos. Nesse momento (e frequentemente antes), os pacientes geralmente são encaminhados a um pneumologista, o qual se espera que seja o principal médico para a resolução desse enigma. Nesse contexto, o teste de exercício cardiopulmonar (TECP), para avaliação de respostas fisiológicas e sensoriais do repouso ao pico do exercício, proporciona uma oportunidade única de desvendar os mecanismos subjacentes à dispneia e as interações desses mecanismos com um amplo espectro de distúrbios. No entanto, o TECP é subutilizado na prática clínica, possivelmente por questões operacionais (custos dos equipamentos, disponibilidade limitada e baixa remuneração) e limitação da formação médica em relação ao método. Para enfrentar esta última deficiência, almejamos fornecer uma estratégia pragmática para a interpretação dos resultados do TECP. O agrupamento dos achados da resposta ao exercício permite a caracterização de padrões que possibilitam ao clínico restringir a lista de possíveis diagnósticos, em vez de apontar uma etiologia específica. Apresentamos uma proposta de avaliação diagnóstica e alguns casos ilustrativos avaliados por TECP. Como a hiper-responsividade das vias aéreas e os distúrbios vasculares pulmonares, que são da competência da pneumologia, são causas comuns de dispneia crônica inexplicada, também objetivamos descrever o papel dos testes de broncoprovocação e o raciocínio diagnóstico para a investigação da circulação pulmonar nesse contexto.
Palavras-chave:
Dispneia; Teste de esforço; Testes de provocação brônquica; Doenças vasculares.
INTRODUCTIONDyspnea is a common and distressing symptom. Population-based studies have shown that the prevalence of mild-to-moderate dyspnea is 9-37% among community-dwelling adults.(1) Higher dyspnea scores were associated with decrements in health status and physical activity in subjects with COPD,(2) and the magnitude of dyspnea can be more discriminating than the functional staging of disease severity (based on FEV1) concerning survival(3) and health-related quality of life. (4) Dyspnea has been shown to be a better predictor of mortality than has angina in patients referred for cardiac stress testing(5) and was associated with a higher risk of mortality when compared with asymptomatic population-based subjects.(6)
There is no definitive classification of dyspnea according to its duration. Dyspnea is usually considered acute when it lasts over hours to days and is considered chronic when it lasts for more than 4 to 8 weeks.(7,8) Multiple conditions can cause chronic dyspnea. Although clinical history taking and physical examination are often insufficient to unequivocally identify the etiology(ies), they remain the mainstays of the diagnostic evaluation, providing guidance to narrow the possibilities and to select appropriate tests. Together with initial examinations, the potential underlying cause(s) can usually be identified in a significant proportion of cases. In two previous seminal studies by Pratter et al.(8,9) investigating chronic dyspnea in clinical practice, approximately half of the participants were considered as having their diagnosis defined based on clinical history taking, physical examination, chest X-ray, and spirometry. Respiratory and cardiac disorders made up the most common etiologies. Dyspnea that remains unexplained after this initial testing sequence represents a major diagnostic challenge. In fact, the cause of dyspnea may remain elusive even after aggressive evaluation.(10,11) Such cases are frequently referred to various different specialists who may conduct the investigation of dyspnea with different-and sometimes contrasting-approaches, and quite often there is no effective communication and collaboration among them. This situation generally results in repeated medical appointments and diagnostic testing, treatments being delayed while the cause of dyspnea is being investigated, and, in the end, it frequently remains "unexplained".(12)
There is no consensus definition, but unexplained dyspnea or dyspnea of unknown origin could be characterized as persistent dyspnea (for at least 3 months) that is clinically and significantly severe (modified Medical Research Council scale score ≥ 2) and remains unexplained after thorough clinical assessment, basic biochemical testing, complete blood count, thyroid function testing, pulmonary function testing, and chest imaging (Figure 1; initial evaluation).(13) Again, although the definition is questionable, the concept of disproportionate or out-of-proportion dyspnea emerges, that is, when a patient has mild abnormalities relative to resting cardiac and pulmonary function testing or imaging findings that do not convincingly explain the severity of dyspnea, at least as expected based on clinical experience and the literature available.(13) Given the epidemiological importance of cardiopulmonary diseases in this context(8,14) and the widespread availability of "advanced" lung function testing, cardiovascular testing, and CT of the chest, it is our impression, based on clinical practice and the literature available,(10-14) that chronic dyspnea is only assumed to be unexplained if the results of those tests are inconclusive (Figure 1; advanced evaluation). In this context, we suggest that HRCT of the chest should be performed using inspiratory/expiratory maneuvers (to detect expiratory dynamic airway collapse, and regional air trapping). Specific studies in this setting are scarce, probably due to the complexity to gather such cases and provide an unequivocal final diagnosis. Those cases account for about 15% of all chronic dyspnea cases and are usually reported in the literature as being treated in multidisciplinary specialized centers.(7,15,16)
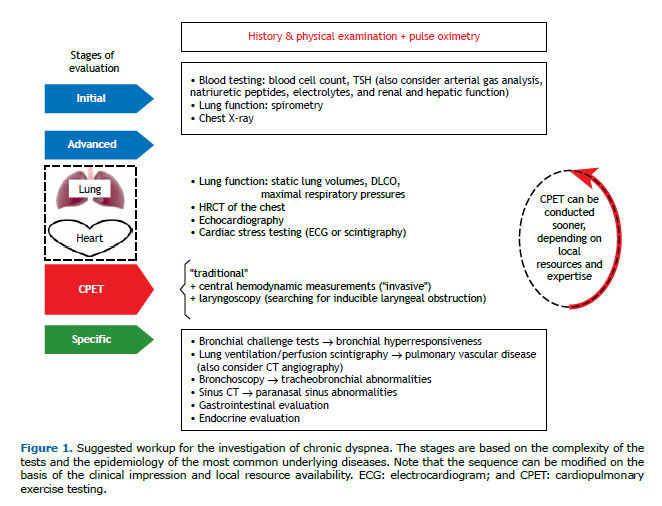
Our intention is not to describe the diagnostic criteria to confirm all potential etiologies of chronic dyspnea. Instead, the overarching objective of the current review is to present the clinical utility of cardiopulmonary exercise testing (CPET) in the evaluation of chronic dyspnea by describing the syndromic patterns related to exercise responses and by indicating a set of different etiological possibilities. Furthermore, given the low specificity and sensitivity of clinical evaluation to detect airway hyperresponsiveness (AHR)(17,18) and pulmonary vascular diseases,(14) the frequency of these conditions causing chronic unexplained dyspnea being high (Table 1), as well as the frequent inconclusive results from spirometry, lung volume measurements, DLCO, and CT of the chest in this context, specific tests to diagnose these conditions should be considered (Figure 1; specific evaluation). Therefore, we also aim to describe the role of bronchial challenge tests (BCTs) and the reasoning for the evaluation of pulmonary circulation in the diagnostic workup to unveil the cause(s) of chronic unexplained or disproportionate dyspnea.
CPETMultiple causes, such as exercise-induced disorders not manifested at rest, exaggerated dyspnea from common conditions (obesity, adverse events of medications), skeletal muscle disease, psychogenic dyspnea (unexplained by organic dysfunction), and rare miscellaneous conditions, should be considered in the face of chronic unexplained dyspnea.(12,13) In other words, chronic unexplained dyspnea might be caused by conditions presenting with greater complexity, unusual presentations of common diseases, or rare disorders, or might even have a psychogenic origin. CPET is a safe procedure that can identify specific physiological abnormalities of the integrated cardiopulmonary, neuromuscular, and sensory systems that contribute to persistent perceived respiratory discomfort. Also of utmost importance is the objective assessment of aerobic capacity and exercise tolerance, as well as of physiological and sensory dynamic responses to exercise. Given the possible dissociation between the perception of dyspnea during activities of daily living and that perception objectively measured during an exercise test,(19) as well as the complex neurophysiology of breathlessness and the influence of psychological and cultural factors,(20) CPET frequently uncovers a preserved exercise capacity and normal responses to exercise, reassuring the patient and the medical team that a relevant organic abnormality is not present. Caution should be considered regarding incipient pathological conditions that lead to a decrement in exercise capacity without reducing peak measurements below the lower limit of normality or that cause abnormal submaximal responses. This can be suspected if a subject whose physical fitness was previously good/excellent complains about exercise intolerance with no evident reason (e.g., reduction or interruption of the physical activity) and is controlled by repeating the CPET (one or more times) during follow-up.
Based on the most common causes of chronic unexplained dyspnea (Table 1),(7-9,10,12) we consider that when the etiology of dyspnea remains elusive after the initial evaluation (Figure 1), and the patient's medical history and physical examination point out to no specific direction, CPET is uniquely poised to unveil the main physiological system(s) that could be related to the perception of dyspnea and to measure exercise tolerance objectively, as well as the perception of dyspnea for a given workload or ventilation, or, in the best scenario, to indicate the absence of physiological impairment. In various circumstances, CPET is not diagnostic per se, but it can uncover patterns of physiological dysfunction, guide further investigation(s), or reassure that no significant cardiopulmonary or other underlying disease is explicit at that moment. Therefore, CPET might guide the investigation and could avoid unnecessary tests and costs. Because there are multiple and combined causes of dyspnea and no cogent scientific evidence to dictate the sequence of evaluation, it seems that good practice follows the clinical impression based on medical history taking, physical examination, and availability of local resources and expertise (Figure 1). After the "supposed" cause(s) of dyspnea is/are identified, it is crucial to consider the response to the treatment(s) employed (e.g., weight loss, exercise training, use of inhaled corticosteroids, etc.), which, if subjectively and objectively effective, reinforces the diagnosis(es) being considered.
CPET is usually performed using a cycle ergometer or a treadmill, although cycling is more frequently used in the setting of chronic respiratory diseases and investigation of dyspnea. The most commonly used protocol includes a resting steady-state period of a few minutes, followed by 2-3 min of unloaded pedaling and, thereafter, a rapidly incremental continuous "ramp" or 1-2-min stepwise increases in the work rate (WR) until exhaustion.(21) Breath-by-breath responses should be recorded and presented in both numerical (tabular report) and graphical formats at average time intervals of 10-20 s.(22) Modern equipment allows routine serial inspiratory capacity (IC) measurements during exercise to track operating lung volumes.(23)
Due to overlapping responses during exercise across disease states, CPET may provide pathognomonic information in rare circumstances; however, it is more realistic to recognize that CPET shortens the list of differential diagnoses. Based on clustering findings, we can derive a syndromic approach indicative of: a) ("normal") physiological responses; b) oxygen delivery/utilization mismatch; c) mechanical ventilatory impairment; d) impaired pulmonary gas exchange/altered ventilatory control; e) obesity; and f) dysfunctional breathing/hyperventilation disorder (Table 2). These patterns of exercise responses must be integrated with the clinical impression based on medical history taking and previous investigations, allowing a specific diagnosis or guiding the next diagnostic steps. (22) Eventually, "conventional" CPET might indicate exercise-related abnormalities; however, it might still be insufficiently discriminative to point out a specific disorder. If metabolic myopathies, exercise-induced pulmonary hypertension (PH), or heart failure with preserved ejection fraction are suspected, invasive CPET (including central hemodynamic assessment via a pulmonary artery catheter and arterial blood gas analysis via a radial catheter) might be indicated.(24) Another less common cause of unexplained chronic dyspnea that can be investigated with an "adapted" CPET is exercise-induced laryngeal obstruction.(25) Exercise protocols vary, but what is crucial is the ability to promote sustained high-intensity efforts for as long as necessary to induce the symptoms. Continuous laryngoscopy during exercise uses a flexible laryngoscope fixed to a head apparatus, which allows real-time visualization of the larynx throughout the study.(25)
Physiological responses to exerciseThe normal response is characterized by ventilatory, circulatory, and metabolic trajectories throughout and at peak exercise within thresholds derived from sedentary healthy populations adjusted for sex and age (Table 3).(18) Table 3 should also be used in order to check abnormalities regarding each parameter, which will allow the characterization of abnormal exercise response patterns described below.
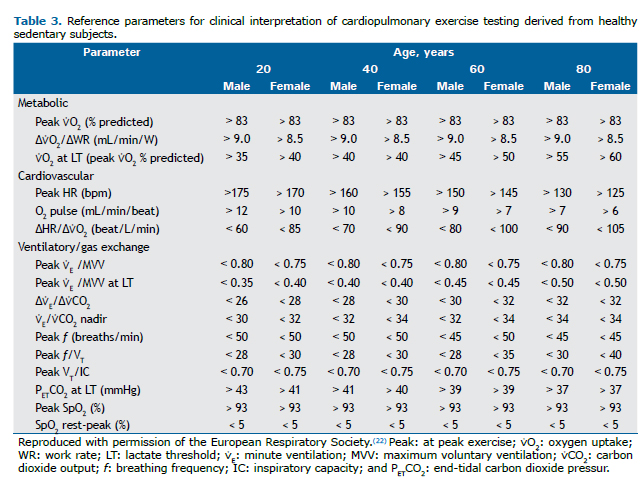
A submaximal test is suspected when no main physiological domain reaches critical values at the end of exercise to constrain exercise continuity(26):
a) metabolic domain: carbon dioxide output (⩒CO2)/oxygen uptake (⩒O2), i.e. respiratory exchange ratio > 1.05; and/or b) cardiovascular domain: HR > 85% of the predicted value; and/or c) ventilatory domain: minute ventilation (⩒E)/maximum voluntary ventilation (MVV) > 0.80-MVV usually being estimated as FEV1 × 35-40-; VT/IC > 0.70(27); and/or end-inspiratory lung volume (EILV)/TLC > 0.9.(28)
CPET is considered "nonphysiologically" limited when none of the above thresholds are reached. In that case, reasons for exercise interruption should be pursued, and CPET should be repeated if the limitation can be relieved: e.g., better hydration for dry throat, analgesics for orthopedic pain, improvement of the seat comfort, familiarization with the cycling equipment, etc.
Oxygen delivery/utilization mismatchThis pattern involves an imbalance between O2 delivery and the energetic needs of the working muscles. Although this pattern implies compromised vascular transport of O2 (reduced cardiac output or convective peripheral O2 delivery in the arterial system) in the majority of the clinical situations, low arterial O2 content and metabolic dysfunction of skeletal muscles (severe sedentariness or myopathies) might produce a similar pattern of responses making it impractical to differentiate between such conditions based on CPET alone. The cluster of findings indicative of this pattern includes:
a) low ⩒O2 at peak exercise (Figure 2A) and at lactate threshold (Figure 2B)-the latter figure demonstrates the noninvasive estimation of the lactate threshold by the V-slope method(29): the inflection in the ⩒CO2 rate of increment as a function of ⩒O2 corresponds to the point where there is a progressive accumulation of lactate as the workload intensifies;
b) a slower increment of ⩒O2 by incremental WR (↓∆⩒O2/∆WR; Figure 2A);
c) low reserve to increase cardiac output at the expense of stroke volume, resulting in an exaggerated in-crease in HR to increments in ⩒O2 (↑∆HR/∆⩒O2) and, consequently, low ⩒O2 for a given HR during submax-imal and peak exercise (↓⩒O2/HR = O2 pulse; Figure 2C)-a flattened or decreasing O2 pulse trajectory during incremental CPET was more commonly found in conditions associated with impaired stroke vol-ume, that is, cardiocirculatory dysfunction.(30,31)
Mechanical ventilatory impairmentConceptually, this pattern occurs when mechanical abnormalities of the interface between the lung and the thorax compromise the adequate ventilation required to a given metabolic demand. The classical approach is to assess how close ventilation is to its ceiling, i.e., measured peak ⩒E in relation to the estimated maximum ventilatory capacity. A rough guide to this maximum is provided by MVV: peak ⩒E/MVV above a certain threshold (Table 2) has been used to indicate "ventilatory limitation" (Figure 3A).(32) However, this ratio correlates poorly with exertional dyspnea in individual subjects with both obstructive and restrictive ventilatory defects. (33) Several dyspneic patients with COPD, mainly those with mild-to-moderate FEV1 reduction,(34) stop exercising and show a preserved ⩒E/MVV ratio, but there is unequivocal evidence of constrained ventilatory mechanics according to parameters of operating lung volumes during exercise. (35) Conversely, a peak ⩒E close to MVV may occur in an otherwise fit subject who can exercise up to high workloads.(36) Therefore, a ⩒E/MVV ratio above the upper limit of normal should be valued to indicate low ventilatory reserve in the context of reduced exercise capacity; however, a high ratio in fit subjects or a low value in subjects with impaired peak ⩒O2 should not be considered as proof of the presence or absence of mechanical ventilatory abnormalities, respectively. There is convincing evidence that dyspnea increases when the mechanical output of respiratory muscles becomes uncoupled from increases in neural respiratory drive.(33,37) Accordingly, indexes of neuromuscular uncoupling have revealed the contribution of impaired ventilation to exertional dyspnea and exercise tolerance better than has peak ⩒E/MVV.(27,28) In practice, neuromechanical coupling is searched by means of serial measurements of VT and IC across the CPET. The difference between EILV and TLC dictates the position of VT on the sigmoid-shaped pressure-volume relationship of the respiratory system. Independently of exercise-induced reduction in IC,(33,38) when the VT/IC ratio reaches approximately 0.7, the EILV/TLC ratio is > 0.9, or the inspiratory reserve volume (IRV), calculated as IRV = IC − VT, is < 0.5-1.0 L below TLC during exercise at relatively low workloads, critical mechanical constraints are present and usually mark the point at which dyspnea abruptly rises toward intolerable levels in subjects with different respiratory diseases.(33,38-41) At this point, there is usually a constraint to VT expansion and, as the exercise continues, any increment in ⩒E happens at the expense of an exaggerated increase in the breathing frequency (f; Figure 3B).
Impaired gas exchange/altered ventilatory controlFrom a practical perspective, these two pathophysiological mechanisms are intrinsically connected, resulting in characteristic responses to exercise. An insufficient decrease in the fraction of breath that is wasted-dead space (VD)-calculated as VD/VT > 0.15-0.20-due to reduced perfusion in relation to alveolar ventilation-ventilation/perfusion (V/Q) mismatch-and/or a low PaCO2 set point at the central control of ventilation results in an increased ⩒E/⩒CO2 ratio, which can be assessed by different metrics (Figure 4).(42) Therefore, the so-called "ventilatory inefficiency" (↑⩒E/⩒CO2) more commonly reflects poor intrapulmonary gas-exchange efficiency.(43) Examples of high ⩒E/⩒CO2 include aging, increased pulmonary artery resistance, pulmonary vascular disorders, congestive heart failure, wasted ventilation in lung diseases-COPD or interstitial lung disease (ILD)-and extraneous sources that activate ventilation (muscle ergoreceptor overactivity, pulmonary C-fiber receptors, or mechanoreceptors), which can exist in the context of all of the abovementioned conditions.(13) Of note, the ⩒E/⩒CO2 slope (i.e., ⩒E plotted as a function of ⩒CO2) increases only if the ventilatory pump is free from mechanical constraints and may even decrease if an obstructive airway disease worsens.(44) Accordingly, caution should be taken not to discard impaired gas exchange in patients with advanced obstructive lung disease and a preserved ⩒E/⩒CO2 slope. In this context, evaluating the intercept of the ⩒E/⩒CO2 slope might represent an alternative for estimating the presence of ventilatory inefficiency.(45)
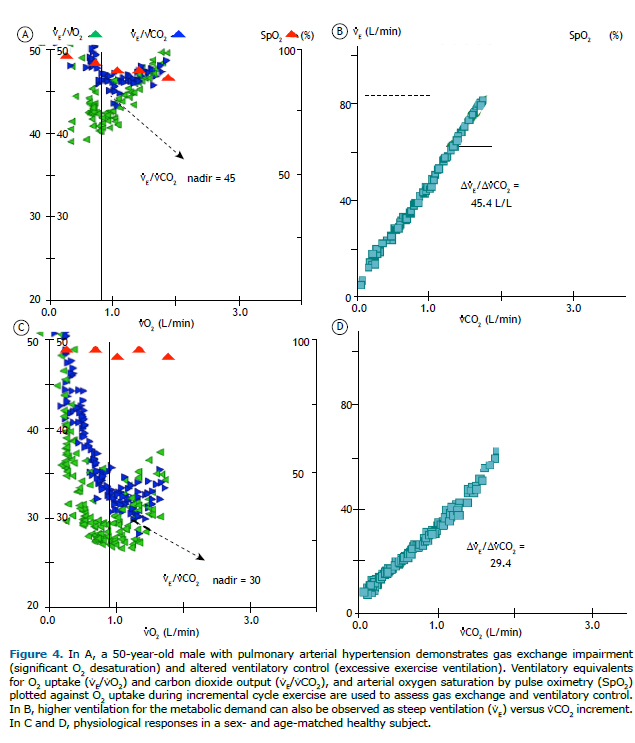
Other findings in patients with significantly impaired gas exchange include exercise-induced hypoxemia (altered PaCO2 in some circumstances) and an enlarged alveolar-arterial O2 tension gradient (> 20 mmHg). Although mild-to-moderate decrements in PaO2 might be missed when oxyhemoglobin saturation is measured by pulse oximetry (SpO2), this is the parameter usually available in practice (Figure 4A). Exertional oxygen desaturation is not a formal feature in healthy subjects unless they are extremely well trained or are exercising at low inspired O2 tension (high altitude). Therefore, SpO2 remains greater than 93% and does not decrease during exercise by more than 4% (Table 3). Low SpO2 (< 88%) stimulates peripheral chemoreceptors and increases inspiratory neural drive and dyspnea. Exercise-related O2 desaturation usually implies disorders with a preponderance of alveoli presenting with low V/Q ratios, which are commonly associated with low mixed venous O2 saturation. Other less common causes are right-to-left shunt and alveolar hypoventilation.(13)
Surprisingly, the major complaint that brings such unexplained cases to medical attention (i.e., dyspnea) is virtually neglected in most international guidelines and clinical laboratories nowadays. Given that CPET measures a multitude of physiological responses that are important for the genesis of dyspnea, it seems natural that special attention should be given to the measurement and interpretation of this symptom. In the absence of mechanical ventilatory constraints, an increased reflex chemostimulation(42) translates into excessive ventilatory response that is proportional to the metabolic demand.(35) Therefore, when an increased drive to breathe can be coupled with the act of ventilating, patients tend to report higher dyspnea for a given workload due to a higher need to ventilate but similar (or slightly increased) dyspnea for the level of ventilation when compared with normal subjects. (46) Conversely, dynamic mechanical ventilatory constraints lead to higher perception of dyspnea as a function of both WR and ventilation (Figure 5).(13,41) Thus, evaluating the intensity of dyspnea as a function of WR and ventilatory demand and compare it with a reference frame obtained from healthy subjects(47) might be useful to discriminate between an increased drive to breathe and mechanical ventilatory impairment as the main pathogenesis of dyspnea.
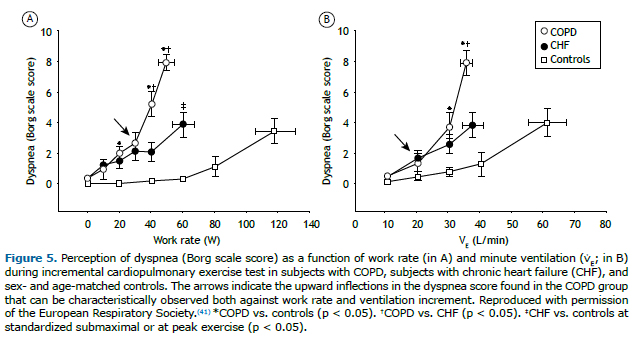
In summary, the presence of impaired gas exchange and altered ventilatory control will infrequently occur in isolation (except during an incipient disorder) from at least one of the other two abnormal patterns-O2 delivery/utilization mismatch and/or mechanical ventilatory impairment. The clinician receiving the CPET results can restrict the list of possible diseases according to the presence of one or more abnormal patterns in conjunction with the whole medical history of the patient available up to that point (13,22):
a) Isolated O2 delivery/utilization mismatch: chronic (systolic or diastolic) heart failure; other cardiocircula-tory abnormalities (ischemic coronary or valve disease); extreme sedentariness; skeletal myopathy; en-docrine/metabolic disorders, and anemia
b) O2 delivery/utilization mismatch plus impaired gas exchange/altered ventilatory control: chronic (systolic or diastolic) heart failure; other cardiocirculatory abnormalities; and pulmonary vascular disease (espe-cially if associated with O2 desaturation)
c) Mechanical ventilatory impairment with or without impaired gas exchange/altered ventilatory control: COPD; other persistent obstructive airway diseases (remodeled asthma, cystic fibrosis, bronchiectasis, or bronchiolitis); exercise-induced obstructive airway disease (asthma), ILD (usually associated with O2 de-saturation); chest wall disease; and isolated respiratory muscle dysfunction
It must be recognized that not all features typical of a given pattern will necessarily be present in every subject and that the final diagnosis of each disease continues being based on defined criteria provided in specific guidelines for each condition.
ObesityObesity represents a unique challenge to ventilatory control during exercise due to the increased metabolic demand to displace a large mass against gravity, the increased work of breathing due to thick chest wall, and altered breathing mechanics.(48) Although such a challenge can go unnoticed in several adapted obese subjects, some can report distressing dyspnea. Despite the fact that the diagnosis of obesity is obvious from resting measurements (height and weight), CPET may be useful to measure exercise capacity and symptoms objectively and to demonstrate the typical exercise response of obese subjects: preserved or even increased aerobic capacity (expressed as % of predicted) despite poor exercise tolerance (low peak WR); exaggerated symptoms; and absence of the abovementioned abnormal exercise response patterns.
The increased metabolic demand (⩒O2 and ⩒CO2) to a given WR is accompanied by proportionally higher cardiovascular and ventilatory responses. The rate of increase in ⩒O2 as a function of WR (∆⩒O2/∆WR), however, remains normal, indicating preserved aerobic efficiency. Starting from a high resting ⩒O2, there is an upward and parallel shift of ⩒O2 as work increases, with peak ⩒O2 reaching normal or near-normal values despite a low peak WR. Due to the increased metabolic demand, obese subjects also tend to report higher leg discomfort and dyspnea for the level of external WR than do nonobese subjects.(22) Finally, the negative effect of reduced chest compliance seems to be counterbalanced by a lower end-expiratory lung volume due to increased intra-abdominal pressure, resulting in a greater volume available for tidal expansion (i.e., IC). Consequently, there is a downward shift in the operating lung volumes and relatively large inspiratory reserve volumes at exercise cessation, which is in contrast to what is observed in the mechanical-ventilatory impairment pattern.(49)
Caution should be taken regarding the obesity hypoventilation syndrome(50) in morbidly obese (BMI > 40 kg/m2)(51) subjects, who may present with exaggerated abnormalities in ventilatory control and mechanical ventilatory response, respectivelyy.
Dysfunctional breathing/hyperventilation disorderLast, but not least, up to one third of the subjects referred for CPET for the investigation of unexplained dyspnea may present with a dysfunctional breathing pattern and/or signals of hyperventilation.(8,10) Albeit not new,(52) given the lack of a formal definition and a gold standard diagnostic method,(53) this condition remains poorly understood and is usually underdiagnosed.(54) Dysfunctional breathing is a wide term describing a group of breathing disorders in subjects with chronic changes in their breathing pattern, resulting in dyspnea and other nonrespiratory symptoms in the absence of, or in excess of, a respiratory disease. Various other terms have been used interchangeably in the literature, including functional breathing disorder, breathing pattern disorder, and behavioral or psychogenic breathlessness. Even though hyperventilation syndrome is often used synonymously with dysfunctional breathing, the former is just one type of the latter, and hyperventilation (i.e., reduced PaCO2) is not necessarily seen in dysfunctional breathing.(53) Before establishing a diagnosis of dysfunctional breathing, organic diseases must be excluded. When common investigations for chronic dyspnea have normal or inconclusive results, CPET seems to be uniquely poised to determine whether breathlessness can be explained (or not) by the presence of any of the aforementioned abnormal exercise response patterns that are indicative of an organic disease. If not, further investigations are required to confirm the presence of dysfunctional breathing (see a detailed description of those methods in this reference).(53) Nevertheless, the exercise responses during CPET can indicate the presence of dysfunctional breathing(22):
a) chaotic breathing, consisting in surges of low and high VT in a background of fast f
b) clear dissociation between ventilation and metabolic demand represented by large variations in ⩒E/⩒CO2 associated with noncyclic fluctuations of end-tidal O2 and CO2 pressures
c) high respiratory exchange ratio at rest (frequently but not always) and a steep ⩒E/⩒CO2 slope
d) high perception of dyspnea for a given WR (a frame of reference for assessing the magnitude of exertion-al dyspnea during incremental cycle ergometry has been recently published),(47) possibly associated with classical symptoms of hyperventilation (tingling, perioral numbness, and dizziness)
Dysfunctional breathing may occur in the context of a coexistent respiratory disease. There is a close link with asthma, but this is less evident regarding COPD and ILD. Therefore, it is important to identify objective evidence of these conditions and optimize treatment as soon as possible before attributing symptoms mainly (or uniquely) to dysfunctional breathing.(53) In addition, close follow-up is recommended in order to investigate the development of commonly associated conditions (especially asthma(55) or other causes of AHR) or some infrequent and difficult-to-diagnose alternative abnormalities (e.g., neuromuscular disease, respiratory muscle weakness,(56,57) and inducible laryngeal obstruction).(25)
BCTs or bronchial provocation testsAHR is a common diagnosis in the context of unexplained dyspnea(9,10,14) and is defined as an increased sensitivity and exaggerated response to non-allergenic stimuli that cause airway narrowing. Although most commonly associated with asthma, AHR is also seen in other obstructive or inflammatory airway diseases, it is common in athletes, and it sometimes occurs in patients with heart failure. (17,58) The magnitude of AHR might increase during exacerbations of underlying diseases, decrease with the use of anti-inflammatory medication, or be absent during asymptomatic periods.(17) BCTs are most often indicated to exclude or confirm the diagnosis of asthma, which can be easily achieved by the clinical history taking, presence of wheezing, and appropriate response to therapy. In this context, reversible airflow obstruction on spirometry is confirmatory. However, spirometry is not always performed when the symptoms are present and may be inconclusive, especially in patients with normal or near-normal lung function. Therefore, BCTs emerge as important tools to unveil AHR as a potential cause of chronic unexplained dyspnea.
In clinical practice, the most common BCTs involve the direct stimulus of the muscarinic receptors on airway smooth muscle using methacholine or the indirect hyperosmolar stimulus due to water loss caused by airway drying and cooling through exercise. (17) In the setting of ongoing clinical symptoms, a negative result of a methacholine BCT, which has higher sensitivity than the indirect method, can be most helpful in making AHR improbable. Exercise BCTs are best indicated when the history of the patient suggests that this type of stimulus triggers clinical complaints. In contrast to the methacholine BCT, exercise BCTs stimulate inflammatory mediators and mechanisms involved in clinical asthma. Therefore, exercise BCTs have higher specificity, but they are less sensitive for the diagnosis of asthma and are preferable when the intention is to confirm asthma, rather than exclude it. Although the recommended exercise protocol to detect AHR is a high-intensity, constant-load test (90% of predicted maximum HR or 60% of MVV for the last 4 min of an overall 6-8 min exercise test),(59) spirometric measurements obtained at 5, 10, 15, and 20 min after a rapid incremental WR protocol (duration: 8-12 min) showed to be as useful in diagnosing AHR in susceptible subjects (≈90% of positive and negative predictive values).(60)
Pulmonary circulation assessmentIn previous studies, pulmonary vascular diseases are causes of unexplained dyspnea in 5-17% of the patients, mostly being secondary to PH or thromboembolic disease (Table 1).(14,61) Those patients often have an unremarkable physical examination. Abnormalities on chest X-rays and on electrocardiograms generally occur only in advanced disease. It is important to highlight that an isolated reduction in DLCO (and normal spirometry) can be a clue for early pulmonary vascular disease when other signs are missing.(62)
The symptoms of PH are nonspecific and mainly related to progressive right ventricular dysfunction. Initial symptoms are typically induced by exercise and include shortness of breath, fatigue, weakness, angina, and syncope, which might be modified by other diseases that cause or are associated with PH as well as by other concurrent diseases.(63) At presentation, almost all patients with PH report dyspnea, which is most often severe and long lasting. In the French National Registry, the majority of the patients with PH had severe symptoms in the initial assessment, 75% of whom presenting with New York Heart Association functional class III or IV, and the delay between the onset of symptoms and the diagnosis was 27 months.(64) Echocardiography, which allows the determination of tricuspid regurgitation velocity and the assessment of other signs of right ventricular dysfunction, is the first test in the diagnostic workup of PH.(65) If the echocardiographic probability of PH is low but clinical suspicion is high, CPET is indicated. In the context of high pre-test probability, O2 delivery/utilization mismatch plus impaired gas exchange is highly suggestive of PH. In the appropriate clinical context, if the echocardiographic probability of PH is intermediate or high, patients should undergo right heart catheterization. Occasionally, some patients may have normal right heart catheterization results at rest, and PH is detected only during invasive CPET, being defined as mean pulmonary arterial pressure ≥ 30 mmHg, cardiac output < 10 L/min, and total pulmonary resistance ≥ 3 Wood units at peak exercise.(66)
In prospective studies, the incidence of chronic thromboembolic pulmonary hypertension (CTEPH) after symptomatic acute pulmonary embolism is reported to range from 0.4% to 6.2%.(67) An important prerequisite to confirm this diagnosis is adequate anticoagulation for at least 3 months. About 75% of the patients with CTEPH have previously confirmed venous thromboembolic event(s). V/Q lung scintigraphy remains the screening test for CTEPH because its accuracy is higher than that of CT angiography. In the future, modern dual-energy CT angiography can become the major test, because it allows the evaluation of anatomical and functional perfusion aspects simultaneously. Different from acute pulmonary embolism with low clinical suspicion when a low D-dimer level has a high negative predictive value, D-dimer alone cannot be used to rule out CTEPH in patients with PH.(68)
Chronic thromboembolic disease (CTED) is characterized by presenting with similar symptoms and perfusion defects similar as to those of CTEPH, but no PH at rest. Exercise intolerance in patients with CTED has been attributed either to exercise-induced PH, showing an increased slope of the pulmonary arterial pressure-flow relationship, or to high dead space ventilation (increased ⩒E/⩒CO2).(69) New/worsened dyspnea and persistent perfusion defects are often encountered after acute pulmonary embolism in 30% and 30-50% of the patients, respectively, hindering the diagnosis of CTED.(70) CPET and echocardiography are recommended to recognize patients in whom symptoms are secondary to (nonvascular) lung disease, left heart disease, obesity, or physical deconditioning. Selected symptomatic patients with CTED may benefit from pulmonary endarterectomy.(71)
Pulmonary arteriovenous malformations are structurally abnormal vessels that provide direct capillary-free communication between pulmonary and systemic circulations (hence, an anatomic right-to-left shunt). Those malformations may be related to hereditary hemorrhagic telangiectasia. Patients complain of dyspnea in 14-51% of the cases.(72) Saline contrast echocardiography is the recommended initial screening test when pulmonary arteriovenous malformations are suspected. The circulatory transit of microbubbles generated by intravenously injected agitated saline contrast is detected by the image of bubbles arriving in the left heart or in the systemic circulation. Positive screening can be confirmed with unenhanced thin-slice (1-2 mm) multidetector CT of the chest and CT pulmonary angiography, being considered the gold standard to confirm the diagnosis. Hepatopulmonary syndrome is another type of intrapulmonary shunt that causes dyspnea, as well as occasional findings of platypnea-orthodeoxia.
Chronic dyspnea is an unusual presentation of pulmonary vasculitis. The clinical picture is more acute and has other clinical and laboratory signs of extrapulmonary manifestations. The diagnosis is made by autoantibody findings and histopathological analysis.(73) Neoplasms of pulmonary vasculature are extremely rare, and the diagnosis can be suspected during the investigation of CTEPH or lung masses.(74)
FINAL CONSIDERATIONSWe showed that CPET can identify physiological abnormalities involving cardiopulmonary, neuromuscular, and sensory systems that can be clustered into patterns of exercise response that are useful for a pragmatic interpretation during the investigation of chronic unexplained or out-of-proportion dyspnea. These clusters of findings should be analyzed in conjunction with the patient's medical history and the results of other complementary tests (i.e., pre-test likelihood of a disease) in order to restrict the list of possible diagnoses, indicate the next step(s), or, hopefully, reach a final diagnosis. In addition, practical issues of BCTs and pulmonary circulation assessment, as well as the spectrum of suspected lung vascular diseases, should be closely acknowledged by pulmonologists dealing with unresolved cases of chronic dyspnea. In selected cases, patients should be evaluated in specialized centers that use advanced/specific methods of investigation.
REFERENCES1. Parshall MB, Schwartzstein RM, Adams L, Banzett RB, Manning HL, Bourbeau J, et al. An official American Thoracic Society statement: update on the mechanisms, assessment, and management of dyspnea. Am J Respir Crit Care Med. 2012;185(4):435-452. https://doi.org/10.1164/rccm.201111-2042ST
2. Gruenberger JB, Vietri J, Keininger DL, Mahler DA. Greater dyspnea is associated with lower health-related quality of life among European patients with COPD. Int J Chron Obstruct Pulmon Dis. 2017;12:937-944. https://doi.org/10.2147/COPD.S123744
3. Nishimura K, Izumi T, Tsukino M, Oga T. Dyspnea is a better predictor of 5-year survival than airway obstruction in patients with COPD. Chest. 2002;121(5):1434-1440. https://doi.org/10.1378/chest.121.5.1434
4. Hajiro T, Nishimura K, Tsukino M, Ikeda A, Oga T, Izumi T. A comparison of the level of dyspnea vs disease severity in indicating the health-related quality of life of patients with COPD. Chest. 1999;116(6):1632-1637. https://doi.org/10.1378/chest.116.6.1632
5. Abidov A, Rozanski A, Hachamovitch R, Hayes SW, Aboul-Enein F, Cohen I, et al. Prognostic significance of dyspnea in patients referred for cardiac stress testing. N Engl J Med. 2005;353(18):1889-1898. https://doi.org/10.1056/NEJMoa042741
6. Frostad A, Søyseth V, Andersen A, Gulsvik A. Respiratory symptoms as predictors of all-cause mortality in an urban community: a 30-year follow-up. J Intern Med. 2006;259(5):520-529. https://doi.org/10.1111/j.1365-2796.2006.01631.x
7. Karnani NG, Reisfield GM, Wilson GR. Evaluation of chronic dyspnea. Am Fam Physician. 2005;71(8):1529-1537.
8. Pratter MR, Curley FJ, Dubois J, Irwin RS. Cause and evaluation of chronic dyspnea in a pulmonary disease clinic. Arch Intern Med. 1989;149(10):2277-2282. https://doi.org/10.1001/archinte.1989.00390100089021
9. Pratter MR, Abouzgheib W, Akers S, Kass J, Bartter T. An algorithmic approach to chronic dyspnea. Respir Med. 2011;105(7):1014-1021. https://doi.org/10.1016/j.rmed.2010.12.009
10. Martinez FJ, Stanopoulos I, Acero R, Becker FS, Pickering R, Beamis JF. Graded comprehensive cardiopulmonary exercise testing in the evaluation of dyspnea unexplained by routine evaluation. Chest. 1994;105(1):168-174. https://doi.org/10.1378/chest.105.1.168
11. Gillespie DJ, Staats BA. Unexplained dyspnea. Mayo Clin Proc. 1994;69(7):657-663. https://doi.org/10.1016/S0025-6196(12)61344-5
12. Huang W, Resch S, Oliveira RK, Cockrill BA, Systrom DM, Waxman AB. Invasive cardiopulmonary exercise testing in the evaluation of unexplained dyspnea: Insights from a multidisciplinary dyspnea center. Eur J Prev Cardiol. 2017;24(11):1190-1199. https://doi.org/10.1177/2047487317709605
13. O'Donnell DE, Milne KM, Vincent SG, Neder JA. Unraveling the Causes of Unexplained Dyspnea: The Value of Exercise Testing. Clin Chest Med. 2019;40(2):471-499. https://doi.org/10.1016/j.ccm.2019.02.014
14. DePaso WJ, Winterbauer RH, Lusk JA, Dreis DF, Springmeyer SC. Chronic dyspnea unexplained by history, physical examination, chest roentgenogram, and spirometry. Analysis of a seven-year experience. Chest. 1991;100(5):1293-1299. https://doi.org/10.1378/chest.100.5.1293
15. Han JN, Zhu YJ, Li SW. Diagnosis and treatment of medically unexplained dyspnea [Article in Chinese]. Zhongguo Yi Xue Ke Xue Yuan Xue Bao. 2004;26(1):76-78.
16. Han JN, Zhu YJ, Li SW, Luo DM, Hu Z, Van Diest I, et al. Medically unexplained dyspnea: psychophysiological characteristics and role of breathing therapy. Chin Med J (Engl). 2004;117(1):6-13.
17. Coates AL, Wanger J, Cockcroft DW, Culver BH; Bronchoprovocation Testing Task Force: Kai-Håkon Carlsen, Diamant Z, et al ERS technical standard on bronchial challenge testing: general considerations and performance of methacholine challenge tests. Eur Respir J. 2017;49(5):1601526. https://doi.org/10.1183/13993003.01526-2016
18. Cockcroft DW, Killian DN, Mellon JJ, Hargreave FE. Bronchial reactivity to inhaled histamine: a method and clinical survey. Clin Allergy. 1977;7(3):235-243. https://doi.org/10.1111/j.1365-2222.1977.tb01448.x
19. Sandberg J, Ekström M. Differences between experienced and recalled breathlessness: a review. Curr Opin Support Palliat Care. 2019;13(3):161-166. https://doi.org/10.1097/SPC.0000000000000448
20. Laviolette L, Laveneziana P; ERS Research Seminar Faculty. Dyspnoea: a multidimensional and multidisciplinary approach. Eur Respir J. 2014;43(6):1750-1762. https://doi.org/10.1183/09031936.00092613
21. Radtke T, Crook S, Kaltsakas G, Louvaris Z, Berton D, Urquhart DS, et al. ERS statement on standardisation of cardiopulmonary exercise testing in chronic lung diseases. Eur Respir Rev. 2019;28(154):180101. https://doi.org/10.1183/16000617.0101-2018
22. Neder JA, Berton DC, Rocha A, Arbex FF, Alencar MC, Degani-Costa LH, et al. Abnormal patterns of response to incremental CPET. In: Palange P, Laveneziana P, Neder JA, Ward SA, editors. Clinical Exercise Testing (ERS Monograph). Lausanne: European Respiratory Society; 2018.
23. Guenette JA, Chin RC, Cory JM, Webb KA, O'Donnell DE. Inspiratory Capacity during Exercise: Measurement, Analysis, and Interpretation. Pulm Med. 2013;2013:956081. https://doi.org/10.1155/2013/956081
24. Maron BA, Cockrill BA, Waxman AB, Systrom DM. The invasive cardiopulmonary exercise test. Circulation. 2013;127(10):1157-1164. https://doi.org/10.1161/CIRCULATIONAHA.112.104463
25. Halvorsen T, Walsted ES, Bucca C, Bush A, Cantarella G, Friedrich G, et al. Inducible laryngeal obstruction: an official joint European Respiratory Society and European Laryngological Society statement. Eur Respir J. 2017;50(3):1602221. https://doi.org/10.1183/13993003.02221-2016
26. Neder JA, Nery LE. Fisiologia clínica do exercício: teoria e prática. São Paulo: Artes Médicas; 2002.
27. Neder JA, Berton DC, Marillier M, Bernard AC, O Donnell DE; Canadian Respiratory Research Network. The role of evaluating inspiratory constraints and ventilatory inefficiency in the investigation of dyspnea of unclear etiology. Respir Med. 2019;158:6-13. https://doi.org/10.1016/j.rmed.2019.09.007
28. Neder JA, Berton DC, Marillier M, Bernard AC, O'Donnell DE; Canadian Respiratory Research Network. Inspiratory Constraints and Ventilatory Inefficiency Are Superior to Breathing Reserve in the Assessment of Exertional Dyspnea in COPD [published correction appears in COPD. 2019 Dec;16(5-6):429]. COPD. 2019;16(2):174-181. https://doi.org/10.1080/15412555.2019.1631776
29. Beaver WL, Wasserman K, Whipp BJ. A new method for detecting anaerobic threshold by gas exchange. J Appl Physiol (1985). 1986;60(6):2020-2027. https://doi.org/10.1152/jappl.1986.60.6.2020
30. Belardinelli R, Lacalaprice F, Carle F, Minnucci A, Cianci G, Perna G, et al. Belardinelli R, Lacalaprice F, Carle F, et al. Exercise-induced myocardial ischaemia detected by cardiopulmonary exercise testing. Eur Heart J. 2003;24(14):1304-1313. https://doi.org/10.1016/S0195-668X(03)00210-0
31. Degani-Costa LH, Nery LE, Rodrigues MT, Gimenes AC, Ferreira EV, Ota-Arakaki JS, et al. Does oxygen pulse trajectory during incremental exercise discriminate impaired oxygen delivery from poor muscle oxygen utilisation?. ERJ Open Res. 2019;5(2):00108-2018. https://doi.org/10.1183/23120541.00108-2018
32. Wasserman K, Whipp BJ. Excercise physiology in health and disease. Am Rev Respir Dis. 1975;112(2):219-249.
33. Faisal A, Alghamdi BJ, Ciavaglia CE, Elbehairy AF, Webb KA, Ora J, et al. Common Mechanisms of Dyspnea in Chronic Interstitial and Obstructive Lung Disorders. Am J Respir Crit Care Med. 2016;193(3):299-309. https://doi.org/10.1164/rccm.201504-0841OC
34. O'Donnell DE, Neder JA, Elbehairy AF. Physiological impairment in mild COPD. Respirology. 2016;21(2):211-223. https://doi.org/10.1111/resp.12619
35. O'Donnell DE, Elbehairy AF, Berton DC, Domnik NJ, Neder JA. Advances in the Evaluation of Respiratory Pathophysiology during Exercise in Chronic Lung Diseases. Front Physiol. 2017;8:82. https://doi.org/10.3389/fphys.2017.00082
36. Dempsey JA, McKenzie DC, Haverkamp HC, Eldridge MW. Update in the understanding of respiratory limitations to exercise performance in fit, active adults. Chest. 2008;134(3):613-622. https://doi.org/10.1378/chest.07-2730
37. Jolley CJ, Luo YM, Steier J, Rafferty GF, Polkey MI, Moxham J. Neural respiratory drive and breathlessness in COPD. Eur Respir J. 2015;45(2):355-364. https://doi.org/10.1183/09031936.00063014
38. Casaburi R, Rennard SI. Exercise limitation in chronic obstructive pulmonary disease. The O'Donnell threshold. Am J Respir Crit Care Med. 2015;191(8):873-875. https://doi.org/10.1164/rccm.201501-0084ED
39. Plachi F, Balzan FM, Gass R, Dorneles RG, Zambiazi R, da Silva DP, et al. Low exertional inspiratory capacity is not related to dynamic inspiratory muscle weakness in heart failure. Respir Physiol Neurobiol. 2018;254:32-35. https://doi.org/10.1016/j.resp.2018.04.005
40. Dorneles R, Plachi F, Gass R, Toniazzo VT, Thome P, Sanches PR, et al. Sensory consequences of critical inspiratory constraints during exercise in pulmonary arterial hypertension. Respir Physiol Neurobiol. 2019;261:40-47. https://doi.org/10.1016/j.resp.2019.01.002
41. Plachi F, Balzan FM, Fröhlich LF, Gass R, Mendes NB, Schroeder E, et al. Exertional dyspnoea-ventilation relationship to discriminate respiratory from cardiac impairment. Eur Respir J. 2020;55(3):1901518. https://doi.org/10.1183/13993003.01518-2019
42. Dempsey JA, Smith CA. Update on Chemoreception: Influence on Cardiorespiratory Regulation and Pathophysiology. Clin Chest Med. 2019;40(2):269-283. https://doi.org/10.1016/j.ccm.2019.02.001
43. Neder JA, Berton DC, Arbex FF, Alencar MC, Rocha A, Sperandio PA, et al. Physiological and clinical relevance of exercise ventilatory efficiency in COPD. Eur Respir J. 2017;49(3):1602036. https://doi.org/10.1183/13993003.02036-2016
44. Neder JA, Arbex FF, Alencar MC, O'Donnell CD, Cory J, Webb KA, et al. Exercise ventilatory inefficiency in mild to end-stage COPD. Eur Respir J. 2015;45(2):377-387. https://doi.org/10.1183/09031936.00135514
45. Neder JA, Di Paolo M, O'Donnell DE, Palange P. On the complexities of measuring exercise "ventilatory efficiency" in obstructive lung diseases. Pediatr Pulmonol. 2020;55(2):280-282. https://doi.org/10.1002/ppul.24556
46. Killian KJ, Summers E, Jones NL, Campbell EJ. Dyspnea and leg effort during incremental cycle ergometry. Am Rev Respir Dis. 1992;145(6):1339-1345. https://doi.org/10.1164/ajrccm/145.6.1339
47. Neder JA, Berton DC, Nery LE, Tan WC, Bourbeau J, O'Donnell DE, et al. A frame of reference for assessing the intensity of exertional dyspnoea during incremental cycle ergometry. Eur Respir J. 2020;56(4):2000191. https://doi.org/10.1183/13993003.00191-2020
48. Babb TG. Obesity: challenges to ventilatory control during exercise--a brief review. Respir Physiol Neurobiol. 2013;189(2):364-370. https://doi.org/10.1016/j.resp.2013.05.019
49. Jensen D, Ofir D, O'Donnell DE. Effects of pregnancy, obesity and aging on the intensity of perceived breathlessness during exercise in healthy humans. Respir Physiol Neurobiol. 2009;167(1):87-100. https://doi.org/10.1016/j.resp.2009.01.011
50. KAUFMAN BJ, FERGUSON MH, CHERNIACK RM. Hypoventilation in obesity. J Clin Invest. 1959;38(3):500-507. https://doi.org/10.1172/JCI103827
51. Chlif M, Temfemo A, Keochkerian D, Choquet D, Chaouachi A, Ahmaidi S. Advanced Mechanical Ventilatory Constraints During Incremental Exercise in Class III Obese Male Subjects. Respir Care. 2015;60(4):549-560. https://doi.org/10.4187/respcare.03206
52. Kerr WJ, Gliebe PA, Dalton JW. Physical Phenomena Associated with Anxiety States: The Hyperventilation Syndrome. Cal West Med. 1938;48(1):12-16.
53. Boulding R, Stacey R, Niven R, Fowler SJ. Dysfunctional breathing: a review of the literature and proposal for classification. Eur Respir Rev. 2016;25(141):287-294. https://doi.org/10.1183/16000617.0088-2015
54. Vidotto LS, Carvalho CRF, Harvey A, Jones M. Dysfunctional breathing: what do we know?. J Bras Pneumol. 2019;45(1):e20170347. https://doi.org/10.1590/1806-3713/e20170347
55. Thomas M, McKinley RK, Freeman E, Foy C, Prodger P, Price D. Breathing retraining for dysfunctional breathing in asthma: a randomised controlled trial. Thorax. 2003;58(2):110-115. https://doi.org/10.1136/thorax.58.2.110
56. Caruso P, Albuquerque AL, Santana PV, Cardenas LZ, Ferreira JG, Prina E, et al. Diagnostic methods to assess inspiratory and expiratory muscle strength. J Bras Pneumol. 2015;41(2):110-123. https://doi.org/10.1590/S1806-37132015000004474
57. Berton DC, Gass R, Feldmann B, Plachi F, Hutten D, Mendes NBS, et al. Responses to progressive exercise in subjects with chronic dyspnea and inspiratory muscle weakness. Clin Resp J. Epub 2020 Aug 16. https://doi.org/10.1111/crj.13265
58. Borges Mde C, Ferraz E, Vianna EO. Bronchial provocation tests in clinical practice. Sao Paulo Med J. 2011;129(4):243-249. https://doi.org/10.1590/S1516-31802011000400008
59. Joos GF, O'Connor B, Anderson SD, Chung F, Cockcroft DW, Dahlén B, et al. Indirect airway challenges [published correction appears in Eur Respir J. 2003 Oct;22(4):718]. Eur Respir J. 2003;21(6):1050-1068. https://doi.org/10.1183/09031936.03.00008403
60. De Fuccio MB, Nery LE, Malaguti C, Taguchi S, Dal Corso S, Neder JA. Clinical role of rapid-incremental tests in the evaluation of exercise-induced bronchoconstriction. Chest. 2005;128(4):2435-2442. https://doi.org/10.1378/chest.128.4.2435
61. Neder JA, Berton DC, Marillier M, Bernard AC, O Donnell DE; Canadian Respiratory Research Network. The role of evaluating inspiratory constraints and ventilatory inefficiency in the investigation of dyspnea of unclear etiology. Respir Med. 2019;158:6-13. https://doi.org/10.1016/j.rmed.2019.09.007
62. Pellegrino R, Viegi G, Brusasco V, Crapo RO, Burgos F, Casaburi R, et al. Pellegrino R, Viegi G, Brusasco V, et al. Interpretative strategies for lung function tests. Eur Respir J. 2005;26(5):948-968. https://doi.org/10.1183/09031936.05.00035205
63. Galiè N, Humbert M, Vachiery JL, Gibbs S, Lang I, Torbicki A, et al. 2015 ESC/ERS Guidelines for the diagnosis and treatment of pulmonary hypertension: The Joint Task Force for the Diagnosis and Treatment of Pulmonary Hypertension of the European Society of Cardiology (ESC) and the European Respiratory Society (ERS): Endorsed by: Association for European Paediatric and Congenital Cardiology (AEPC), International Society for Heart and Lung Transplantation (ISHLT) [published correction appears in Eur Respir J. 2015 Dec;46(6):1855-6]. Eur Respir J. 2015;46(4):903-975. https://doi.org/10.1183/13993003.01032-2015
64. Humbert M, Sitbon O, Chaouat A, Bertocchi M, Habib G, Gressin V, et al. Pulmonary arterial hypertension in France: results from a national registry. Am J Respir Crit Care Med. 2006;173(9):1023-1030. https://doi.org/10.1164/rccm.200510-1668OC
65. Kovacs G, Dumitrescu D, Barner A, Greiner S, Grünig E, Hager A, et al. Definition, clinical classification and initial diagnosis of pulmonary hypertension: Updated recommendations from the Cologne Consensus Conference 2018. Int J Cardiol. 2018;272S:11-19. https://doi.org/10.1016/j.ijcard.2018.08.083
66. Naeije R, Saggar R, Badesch D, Rajagopalan S, Gargani L, Rischard F, et al. Exercise-Induced Pulmonary Hypertension: Translating Pathophysiological Concepts Into Clinical Practice. Chest. 2018;154(1):10-15. https://doi.org/10.1016/j.chest.2018.01.022
67. Kim NH, Delcroix M, Jais X, Madani MM, Matsubara H, Mayer E, et al. Chronic thromboembolic pulmonary hypertension. Eur Respir J. 2019;53(1):1801915. https://doi.org/10.1183/13993003.01915-2018
68. Arunthari V, Burger CD. Utility of d-dimer in the diagnosis of patients with chronic thromboembolic pulmonary hypertension. Open Respir Med J. 2009;3:85-89. https://doi.org/10.2174/1874306400903010085
69. van Kan C, van der Plas MN, Reesink HJ, van Steenwijk RP, Kloek JJ, Tepaske R, et al. van Kan C, van der Plas MN, Reesink HJ, et al. Hemodynamic and ventilatory responses during exercise in chronic thromboembolic disease. J Thorac Cardiovasc Surg. 2016;152(3):763-771. https://doi.org/10.1016/j.jtcvs.2016.05.058
70. Klok FA, van Kralingen KW, van Dijk AP, Heyning FH, Vliegen HW, Huisman MV. Prevalence and potential determinants of exertional dyspnea after acute pulmonary embolism. Respir Med. 2010;104(11):1744-1749. https://doi.org/10.1016/j.rmed.2010.06.006
71. Taboada D, Pepke-Zaba J, Jenkins DP, Berman M, Treacy CM, Cannon JE, et al. Outcome of pulmonary endarterectomy in symptomatic chronic thromboembolic disease. Eur Respir J. 2014;44(6):1635-1645. https://doi.org/10.1183/09031936.00050114
72. Shovlin CL. Pulmonary arteriovenous malformations. Am J Respir Crit Care Med. 2014;190(11):1217-1228. https://doi.org/10.1164/rccm.201407-1254CI
73. Nasser M, Cottin V. The Respiratory System in Autoimmune Vascular Diseases. Respiration. 2018;96(1):12-28. https://doi.org/10.1159/000486899
74. Restrepo CS, Betancourt SL, Martinez-Jimenez S, Gutierrez FR. Tumors of the pulmonary artery and veins. Semin Ultrasound CT MR. 2012;33(6):580-590. https://doi.org/10.1053/j.sult.2012.04.002