ABSTRACT
Objective: Brain death (BD) triggers important hemodynamic and inflammatory alterations, compromising the viability of organs suitable for transplantation. To better understand the mi-crocirculatory alterations in donor lungs caused by BD. The present study investigated the pul-monary microcirculation in a rodent model of BD via intravital microscopy. Methods: Male Wistar rats were anaesthetized and mechanically ventilated. They were trepanned and BD was induced through the increase in intracranial pressure. As control group, sham-operated (SH) rats were trepanned only. In both groups, expiratory O2 and CO2 were monitored and after three hours, a thoracotomy was performed, and a window was created to observe the lung surface using an epi-fluorescence intravital microscopy. Lung expression of intercellular adhesion molecule (ICAM)-1 and endothelial nitric oxide synthase (eNOS) was evaluated by immunohistochemistry, and cytokines were measured in lung samples. Results: Three hours after the surgical proce-dures, pulmonary perfusion was 73% in the SH group. On the other hand, BD animals showed an important decrease in organ perfusion to 28% (p = 0.036). Lung microcirculatory compromise after BD induction was associated with an augmentation of the number of leukocytes recruited to lung tissue, and with a reduction in eNOS expression and an increase in ICAM-1 expression on lung endothelial cells. BD rats showed higher values of expiratory O2 and lower values of CO2 in comparison with SH animals after three hours of monitoring. Conclusion: Data presented showed that BD triggers an important hypoperfusion and inflammation in the lungs, compromis-ing the donor pulmonary microcirculation
Keywords:
Brain death; Inflammation; Intravital microscopy; Lung microcirculation.
RESUMO
Objetivo: A morte cerebral (MC) desencadeia alterações hemodinâmicas e inflamatórias importantes, comprometendo a viabilidade dos órgãos empregados em transplantes. Para compreender melhor as alterações microcirculatórias nos pulmões de doadores com MC, o presente estudo investigou a microcirculação pulmonar em um modelo de roedor com MC via microscopia intravital. Métodos: Ratos Wistar machos foram anestesiados e ventilados mecanicamente. Eles foram submetidos a trepanação e a MC induzida por meio do aumento da pressão intracraniana. Os ratos do grupo Sham (SH), utilizado como controle, foram submetidos apenas à trepanação. Em ambos os grupos, foram monitorados o O2 expiratório e o CO2, e, após 3 horas, foi realizada a toracotomia e criada uma janela para observar a superfície pulmonar usando o sistema de microscopia intravital. As expressões pulmonares das moléculas de adesão intercelular (ICAM)-1 e da óxido nítrico-sintase endotelial (eNOS) foram avaliadas por imuno-histoquímica, e as citocinas foram medidas em amostras pulmonares. Resultados: Três horas após os procedimentos cirúrgicos, a perfusão pulmonar foi de 73% no grupo SH. Por outro lado, os animais com MC apresentaram uma importante diminuição na perfusão do órgão para 28% (p = 0,036). O comprometimento microcirculatório pulmonar após a indução de MC foi associado a um aumento do número de leucócitos recrutados para o tecido pulmonar, além de uma redução na expressão de eNOS e um aumento na expressão de ICAM-1 nas células endoteliais do pulmão. Os ratos com MC apresentaram valores mais elevados de O2 expiratório e valores mais baixos de CO2 em comparação com os animais SH após 3 horas de monitorização. Conclusões: Os dados apresentados demonstraram que a MC desencadeia uma importante hipoperfusão e inflamação nos pulmões, comprometendo a microcirculação pulmonar do doador.
Palavras-chave:
Morte encefálica; Inflamação; Microscopia intravital; Microcirculação pulmonar.
INTRODUCTIONLung transplantation continues to be restricted by limited long-term survival and high incidence of lung donor impairment. Several studies have shown that microvascular damage seems to be an important cause of early and long-term lung graft failure,(1,2) like other solid organs,(3,4) thus stressing the importance of preserving a functional microvasculature as a therapeutic strategy for preventing chronic fibrotic remodeling.
Besides alloimmune inflammation and ischemia reperfusion injury, which are considered as the causes of microvascular injury in the solid organs used for transplantation,(1-4) pathophysiological changes triggered by brain death (BD) also can adversely affect the microcirculatory system. In a previous study,(5) observation of rat mesenteric microcirculation in situ and in vivo by intravital microscopy showed that BD triggers a persistent mesenteric hypoperfusion, local inflammation, and organ dysfunction. To better understand the microcirculatory alterations in the BD donor lungs, the present study aimed to investigate pulmonary microcirculation in a rodent model of BD via intravital microscopy.
METHODSExperimental groups and surgical proceduresWe used male Wistar rats (300±30 g) divided in two groups: BD group (n=10), rats in which BD was induced; sham-operated group (n=10), animals subjected to the same surgical process without BD induction. Each group included five rats for intravital microscopy analysis and other five per group were used for lung tissue analysis to avoid any bias related to the invasive lung study. All rats received humane care in compliance with the ethical principles adopted by the Brazilian College of Animal Experimentation. The protocol was approved by the Faculdade de Medicina of the Universidade de Sao Paulo Ethics Committee for Research Projects Analysis.
The anesthesia was induced in a chamber with 5% of isoflurane, followed by intubation and mechanical ventilation (Harvard Apparatus, USA) with tidal volume of 10 mL/kg, frequency of 70 breaths/min and FiO2 of 100%. The ventilator was connected to a gas analyzer (AD Instruments, Australia) and the percentage of exhaled O2 and CO2 was measured in all animals during the experiment.
Brain death modelSurgical procedures to BD induction were performed as previously described.(5) BD was induced by a Fogarty-4F catheter placed into intracranial cavity and suddenly inflated with 0.5 mL of saline solution; then, anesthesia was immediately interrupted. The sudden increase in intracranial pressure was confirmed by hypertensive episode, maximal pupil dilatation and absence of reflex, according to a previously described protocol.(5) Sham-operated rats, submitted to trepanation only, were used as control group.
Before trepanation, the left carotid artery was cannulated, and a catheter was placed into the vessel for continuous monitoring of mean arterial blood pressure in rats of both groups and draw blood samples. As previously observed in other experimental models, this procedure does not lead to important cerebral hemispheric ischemia.(6) The jugular vein was cannulated for continuous infusion of 0.9% saline solution (2 mL/h) to minimize dehydration.
Intravital microscopy studyThree hours after BD induction, a right thoracotomy was performed in five animals per group, creating a window for in vivo microscopic examination of the lung tissue. Pulmonary microcirculation was evaluated using an epifluorescence intravital microscopy system (Carl Zeiss, Germany). To evaluate lung perfusion, fluorescein isothiocyanate (FITC) conjugated to albumin was administered to the rats by a catheter placed into the jugular vein. The number of microvessels with a diameter of less than 30 µm with or without blood flow were determined in an area of 0.2 mm2, which were calculated using the AxioVision LE software. After intravenous injection with rhodamine 6G, the number of adhered or migrated leukocytes was determined in the same area of the lung tissue. For better resolution, the ventilation was performed with positive end-expiratory pressure (PEEP) of 5 cm H2O during the intravital microscopy analyses. During video recording the animals were maintained in apnea at the end of the maximal inspiration. All images were recorded using 20 x objective lens.
Immunohistochemistry analysesAfter the euthanasia, the lungs of the other five animals per group were removed, insufflated with OCT solution (Tissue Teck, USA), immersed in hexane and frozen with liquid nitrogen. The expression levels of intercellular adhesion molecule (ICAM)-1 and endothelial nitric oxide synthase (eNOS) on the endothelium of pulmonary microvessels were determined by immunohistochemistry. Cryosections of lungs (8 µm) were fixed in cold acetone for 10 min. The slides were washed with TRIS buffer-saline tween-20 (TBS-T) solution and the nonspecific binding sites were blocked using TBS-T containing 1% bovine serum albumin (BSA), and the endogenous peroxidase was blocked using 2% H2O2 solution. The sections were incubated for 1 hour at 37 °C with an anti-rat ICAM-1 antibody (Santa Cruz, USA) or with an anti-eNOS antibody (Abcam, USA). After incubation, the slide glasses were washed with TBS-T and incubated with horseradish peroxidase (HRP) secondary antibodies (Millipore, USA). Horseradish peroxidase substrate (3-amino-9-ethylcarbazole; Vector Laboratories, USA) was used for staining, and 3-amino-9-ethylcarbazole-stained objects in vessels walls were identified after threshold determination. The staining area fractions were quantified using an image analyzer (NIS-elements; Nikon, Japan). The background reaction was determined in lung sections incubated in the absence of primary antibody (negative control)
Tissue concentrations of cytokinesThe lung tissue levels of tumor necrosis factor (TNF)-α, interleukin (IL)-1β, IL-6, and IL-10 were quantified by enzyme-linked immunosorbent assay (ELISA; R&D Systems, USAR&D Systems Inc). A fragment of the frozen harvested lungs was sectioned, immersed in saline solution and milled in a tissue mixer (Miltenyi Biotec, USA). The supernatant was analyzed by ELISA as recommended by the manufacturer. The results were expressed as ng/g or pg/gtissue.
Statistical analysesThe sample size was based on similar studies previously performed by our group.(5,7) All data are presented as mean±SEM (standard error of the mean). The results between groups were compared by a non-parametric Mann-Whitney test using Graph Pad Prism software version 6.1. A p value less than 0.05 was considered significant.
RESULTSThere were no deaths and the overall volume of fluid administered during the experiments was similar between the groups. As expected, BD induced a hypertensive episode sudden after the increase in intracranial pressure followed by hypotension, whereas in SH rats the mean arterial blood pressure did not change over time. The hypertensive episode is a peculiar feature in BD and confirms that the model is well established.
Microvascular pulmonary perfusion was evaluated after 3 hours of surgical procedures by epifluorescence microscopy. As illustrated in Figure 1, BD rats showed a significant decrease in the percentage of perfused microvessels compared with SH rats in an area of 0.2 μm2. The pulmonary hypoperfusion was associated with increased leukocyte recruitment to the lung tissue as illustrated in Figure 2. We observed a higher number of recruited leukocytes to the lungs in BD rats when compared with SH group.
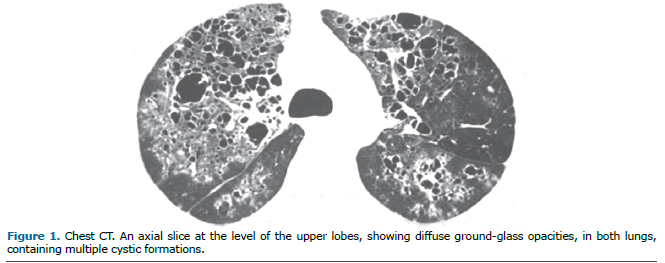
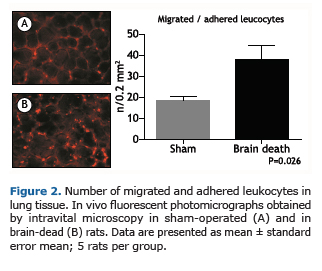
The endothelial cells of pulmonary microvessels in BD rats showed augmentation in the expression of ICAM-1 in comparison with SH group. In contrast, BD presented lower levels of eNOS expression on the endothelium (Figure 3A and B). Table 1 shows the values of tissue cytokines levels in the pulmonary tissue of both groups. We observed no differences in pulmonary IL-1β, IL-6 and IL-10 concentrations between groups, but TNF-α concentration increased significantly in BD group in comparison with SH animals.
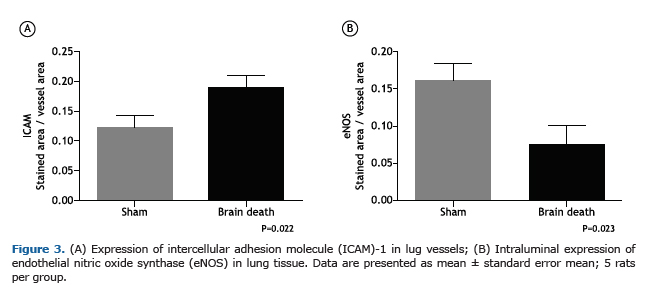
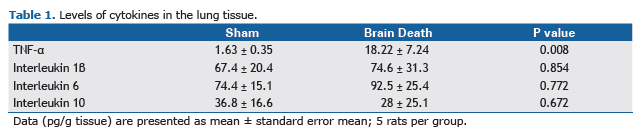
Before BD induction, partial pressure of arterial oxygen and carbon dioxide were similar in both groups. At the end of the experiment, PaO2 in the BD group (204.5 ± 35.07 mmHg) was lower than in SH group (303.4 ± 26.47 mmHg, p=0.039). There were no significant differences in PaCO2 values between the groups (SH, 31.66 ± 3.81; BD, 37.68 ± 2.64 mmHg, p=0.235). The expiratory gases were measured throughout the experiment in animals of both groups. After three hours of monitoring, BD rats showed higher values of expiratory O2 and lower values of CO2 in comparison with SH animals at the same time point (Figure 4A and B).
DISCUSSIONThe elucidation of the pathophysiological events triggered by BD may lead to a better treatment of organ donors and, consequently, a better outcome after transplantation. Several studies have been performed to clarify these events, either in clinical or experimental models. Notwithstanding, the observation of pulmonary microcirculation in vivo and in situ was not performed in brain dead lung donor before this study and, herein, we demonstrated that BD was responsible for a pulmonary hypoperfusion with an increase in the leukocyte recruitment to the lungs. These microcirculatory changes were associated with endothelial alterations such as augmentation in the expression of ICAM-1 and decrease in eNOS expression. Finally, these observations were correlated with alterations on the percentage of exhaled O2 and CO2, indicating that BD was responsible for an impairment on donor lung function, despite the absence of blood gases compromise due to the short period of observation.
Regarding lung intravital microscopic data, Ivanov and co-workers describe that the alveolus is a spherical body surrounded by a microvascular network.(7) On the other hand, Sack et al.(2) showed that pulmonary microcirculation exhibits a significant number of no-reflow areas with extremely reduced red blood cell velocities after lung transplantation in pigs, situation that was probably triggered by the ischemia-reperfusion injury. The analogous observation regarding the lung microcirculation documented in the current study after BD induction indicates another cause to this phenomenon and reinforces the relevance of microvascular injury since the donor management.
The correlation between BD and microcirculation compromise was previously verified in abdominal organs. It was shown that BD leads to a decreased organ perfusion in mesenteric, pancreatic and hepatic microcirculation and that these changes were associated with increased local inflammation.(5,8-10) The described events started early after BD induction and may be clearly observed three hours after brain damage.(5,8) Similarly, the increase of leukocyte recruitment to the lungs was also observed in the current study after BD induction, as a cascade of events that includes margination, rolling, adhesion, crawling and transmigration to perivascular tissue.(11)
The microvasculature has an important role in the long-term health of transplanted organs, and the mechanisms of microvascular damage after transplantation are mainly related to the endothelial cells injury by oxidative stress and immune response associated factors.(1-4,12) Oxidative stress is normally perceived in solid organ transplants and has been attributable to several factors including ischemia-reperfusion injury, post-transplant graft dysfunction, immunosuppressive drugs, as well as primary illness of the transplanted organ that has BD as its most important cause.
During oxidative stress augmentation, alloantibodies may induce endothelium cells death by complement dependent mechanisms, which stimulate endothelium cells expression of adhesion molecules. Endothelial cells apoptosis via suppression of eNOS can be also observed.(12) The current documentation that these mechanisms are also directly involved in the lung microvascular compromise induced by BD, clearly demonstrated that microvascular damage initiates before the transplantation process, adding a worse substrate for the subsequent changes due to ischemia-reperfusion injury and immunological alterations.
The inflammation observed in BD rats was also characterized by an increase in ICAM-1 expression on lungs, demonstrating the interaction between leukocytes and endothelial cells.(11) In a previous study, the augmentation in the expression of the adhesion molecules was associated with an important reduction in the serum corticosterone levels.(5) It is well known that BD interrupts the hormonal axis leading to a reduction in the release of hormones, including glucocorticoids, such as corticosterone, which act controlling the inflammation and avoiding the overexpression of adhesion molecules on the endothelial cells.(5)
Other studies demonstrated that BD leads to an augmentation in the serum level of cytokines, adhesion molecules expression, and leukocyte recruitment to perivascular tissue,(10,13-17) suggesting a connection among these features. This study showed that rats with BD exhibited increased serum levels of TNF-α in comparison to SH animals in the lung tissue, despite the absence of differences between the other investigated cytokines. This observation was similarly observed in a previous study with the same protocol, suggesting that some of the observed inflammatory events are triggered by BD-associated trauma.(5)
From these findings, we can conclude that BD triggers an important hypoperfusion in the lungs, compromising the pulmonary microcirculation and function, once leukocyte recruitment associated with endothelial activation is observed. During the different phases of the lung transplantation process, including the management of BD organ donors, extensive microvascular injury with insufficient repair leads to pathogenic angiogenesis and subsequent fibrosis. Therefore, the preservation of a healthy microvasculature by inhibiting pathways that lead to microvascular injury is a fundamental strategy to improve both lung donor availability and graft survival extension.
REFERENCES1. Nicolls MR, Hsu JL, Jiang X. Microvascular injury after lung transplantation. Curr Opin Organ Transplant. 2016;21(3):279-84. http://dx.doi.org/10.1097/MOT.0000000000000307. PMid:26967995.
2. Sack FU, Dollner R, Reidenbach B, Koch A, Gebhard MM, Hagl S. Intravital microscopy of pulmonary microcirculation after single lung transplantation in pigs. Transplant Proc. 2006;38(3):737-40. http://dx.doi.org/10.1016/j.transproceed.2006.01.060. PMid:16647459.
3. Jiang X, Sung YK, Tian W, Qian J, Semenza GL, Nicolls MR. Graft microvascular disease in solid organ transplantation. J Mol Med. 2014;92(8):797-810. http://dx.doi.org/10.1007/s00109-014-1173-y. PMid:24880953.
4. Labarrere CA, Jaeger BR, Kassab GS. Cardiac allograft vasculopathy: microvascular arteriolar capillaries ('capioles") and survival. Front Biosci. 2017;9(1):110-28. http://dx.doi.org/10.2741/e790. PMid:27814594.
5. Simas R, Sannomiya P, Cruz JW, Correia CJ, Zanoni FL, Kase M, et al. Paradoxical effects of brain death and associated trauma on rat mesenteric microcirculation: an intravital microscopic study. Clinics. 2012;67(1):69-75. http://dx.doi.org/10.6061/clinics/2012(01)11. PMid:22249483.
6. Ivanov KP. Circulation in the lungs and microcirculation in the alveoli. Respir Physiol Neurobiol. 2013;187(1):26-30. http://dx.doi.org/10.1016/j.resp.2013.02.022. PMid:23466953.
7. Simas R, Ferreira SG, Menegat L, Zanoni FL, Correia CJ, Silva IA, et al. Mesenteric hypoperfusion and inflammation induced by brain death are not affected by inhibition of the autonomic storm in rats. Clinics. 2015;70(6):446-52. http://dx.doi.org/10.6061/clinics/2015(06)11. PMid:26106965.
8. Cavalcante LP, Ferreira SG, Pereira DR, Moraes SR, Simas R, Sannomiya P, et al. Acute administration of oestradiol or progesterone in a spinal cord ischaemia-reperfusion model in rats. Interact Cardiovasc Thorac Surg. 2018;26(2):196-201. http://dx.doi.org/10.1093/icvts/ivx314. PMid:29049608.
9. Obermaier R, Dobschuetz E, Keck T, Hopp H-H, Drognitz O, Schareck W, et al. Brain death impairs pancreatic microcirculation. Am J Transplant. 2004;4(2):210-5. http://dx.doi.org/10.1046/j.1600-6143.2003.00317.x. PMid:14974941.
10. Yamagami K, Hutter J, Yamamoto Y, Schauer RJ, Enders G, Leiderer R, et al. Synergistic effects of brain death and liver steatosis on the hepatic microcirculation. Transplantation. 2005;80(4):500-5. http://dx.doi.org/10.1097/01.tp.0000167723.46580.78. PMid:16123725.
11. Leick M, Azcutia V, Newton G, Luscinskas FW. Leukocyte recruitment in inflammation: basic concepts and new mechanistic insights based on new models and microscopic imaging technologies. Cell Tissue Res. 2014;355(3):647-56. http://dx.doi.org/10.1007/s00441-014-1809-9. PMid:24562377.
12. Jiang X, Khan MA, Tian W, Beilke J, Natarajan R, Kosek J, et al. Adenovirus-mediated HIF-1 gene transfer promotes repair of mouse airway allograft microvasculature and attenuates chronic rejection. J Clin Invest. 2011;121(6):2336-49. http://dx.doi.org/10.1172/JCI46192. PMid:21606594.
13. Barklin A. Systemic inflammation in the brain-dead organ donor. Acta Anaesthesiol Scand. 2009;53(4):425-35. http://dx.doi.org/10.1111/j.1399-6576.2008.01879.x. PMid:19226294.
14. Van der Hoeven JA, Molema G, Ter Horst GJ, Freund RL, Wiersema J, van Schilfgaarde R, et al. Relationship between duration of brain death and hemodynamic (in)stability on progressive dysfunction and increased immunologic activation of donor kidneys. Kidney Int. 2003;64(5):1874-82. http://dx.doi.org/10.1046/j.1523-1755.2003.00272.x. PMid:14531823.
15. Van Der Hoeven JA, Ter Horst GJ, Molema G, de Vos P, Girbes AR, Postema F, et al. Effects of brain death and hemodynamic status on function and immunologic activation of the potential donor liver in the rat. Ann Surg. 2000;232(6):804-13. http://dx.doi.org/10.1097/00000658-200012000-00009. PMid:11088075.
16. Skrabal CA, Thompson LO, Potapov EV, Southard RE, Joyce DL, Youker KA, et al. Organ-specific regulation of pro-inflammatory molecules in heart, lung, and kidney following brain death. J Surg Res. 2005;123(1):118-25. http://dx.doi.org/10.1016/j.jss.2004.07.245. PMid:15652959.
17. Rostron AJ, Avlonitis VS, Cork DMW, Grenade DS, Kirby JA, Dark JH. Hemodynamic resuscitation with argi-nine vasopressin reduces lung injury after brain death in the transplant donor. Transplantation. 2008;85(4):597-606. http://dx.doi.org/10.1097/TP.0b013e31816398dd. PMid:18347540.