ABSTRACT
Objective: To evaluate the effects that early and late systemic administration of methylprednisolone have on lungs in a rat model of brain death. Methods: Twenty-four male Wistar rats were anesthetized and randomly divided into four groups (n = 6 per group): sham-operated (sham); brain death only (BD); brain death plus methylprednisolone (30 mg/kg i.v.) after 5 min (MP5); and brain death plus methylprednisolone (30 mg/kg i.v.) after 60 min (MP60). In the BD, MP5, and MP60 group rats, we induced brain death by inflating a balloon catheter in the extradural space. All of the animals were observed and ventilated for 120 min. We determined hemodynamic and arterial blood gas variables; wet/dry weight ratio; histological score; levels of thiobarbituric acid reactive substances (TBARS); superoxide dismutase (SOD) activity; and catalase activity. In BAL fluid, we determined differential white cell counts, total protein, and lactate dehydrogenase levels. Myeloperoxidase activity, lipid peroxidation, and TNF-α levels were assessed in lung tissue. Results: No significant differences were found among the groups in terms of hemodynamics, arterial blood gases, wet/dry weight ratio, BAL fluid analysis, or histological score-nor in terms of SOD, myeloperoxidase, and catalase activity. The levels of TBARS were significantly higher in the MP5 and MP60 groups than in the sham and BD groups (p < 0.001). The levels of TNF-α were significantly lower in the MP5 and MP60 groups than in the BD group (p < 0.001). Conclusions:áIn this model of brain death, the early and late administration of methylprednisolone had similar effects on inflammatory activity and lipid peroxidation in lung tissue.
RESUMO
Objetivo: Avaliar os efeitos da administração sistêmica precoce e tardia de metilprednisolona nos pulmões em um modelo de morte encefálica em ratos. Métodos: Vinte e quatro ratos Wistar machos foram anestesiados e randomizados em quatro grupos (n = 6 por grupo): sham, somente morte encefálica (ME), metilprednisolona i.v. (30 mg/kg) administrada 5 min após a morte encefálica (MP5) e 60 min após a morte encefálica (MP60). Os grupos ME, MP5 e MP60 foram submetidos à morte encefálica por insuflação de um balão no espaço extradural. Todos os animais foram observados e ventilados durante 120 min. Foram determinadas variáveis hemodinâmicas e gasométricas, relação peso úmido/seco, escore histológico, thiobarbituric acid reactive substances (TBARS, substâncias reativas ao ácido tiobarbitúrico), atividade de superóxido dismutase (SOD) e de catalase, assim como contagem diferencial de células brancas, proteína total e nível de desidrogenase lática no LBA. A atividade da mieloperoxidase, peroxidação lipídica e níveis de TNF- foram avaliados no tecido pulmonar. Resultados: Não foram observadas diferenças significativas nas variáveis hemodinâmicas e gasométricas, relação peso úmido/seco, análises do LBA, escore histológico, SOD, mieloperoxidase e catalase entre os grupos. Os níveis de TBARS foram significativamente maiores nos grupos MP5 e MP60 do que nos grupos sham e ME (p < 0,001). Os níveis de TNF- foram significativamente menores nos grupos MP5 e MP60 do que no grupo ME (p < 0,001). Conclusões: Neste modelo de morte cerebral, a administração precoce e tardia de metilprednisolona apresentou efeitos semelhantes sobre a inflamação e a peroxidação lipídica no tecido pulmonar.
Palavras-chave:
Ratos; Morte encefálica; Estresse oxidativo; Pulmão; Hidroxicorticosteroides.
IntroductionLung transplantation is an established therapeutic option for patients with end-stage lung disease. In the last decade, there has been a significant increase in the number of lung transplant centers.(1) As a result, the number of patients on waiting lists has been increasing. In contrast, the donor pool has remained fairly constant, the proportion of available lungs that are suitable for transplantation ranging from 4.9% to 27%.(1-4) Recently, with the use of lungs from marginal donors and the encouraging results of ex vivo lung perfusion for lung reconditioning, there has been a trend toward an increase in the number of lung transplants.(5,6)
Brain-dead donors still represent the major source of organs for transplantation. In the ICU, the lungs of potential donors are exposed to direct damage caused by the process related to the occurrence of brain death. In addition, the lungs are at risk for acute lung injury secondary to trauma, prolonged mechanical ventilation, transfusion, ischemia, aspiration, and infection.(7,8)
Brain death is defined as the death of all central neurological tissue, resulting in the loss of cerebral function.(9) The pathophysiology of brain death is complex, involving sympathetic, hemodynamic, and inflammatory mechanisms that can injure the lung.(7,10) Significant endocrine changes result in a decrease in the plasma levels of adrenocorticotropic hormone, cortisol, triiodothyronine, thyroxine, insulin, and vasopressin.(7)
Currently, there is a consensus that organ grafts should not be considered immunologically inert. Donor risk factors, such as previous diseases, age, cause of death, donor management, and, most importantly, brain death itself, can reprogram the graft, making it an immunologically active organ.(8,11) New strategies for the treatment of potential brain-dead donors represent a promising approach to reducing the immunogenicity of the graft and improving the quality of the organ before transplantation.(12)
The administration of systemic corticosteroids to brain-dead donors is known to be beneficial mostly because of its ability to modulate the systemic inflammatory response caused by brain death. This modulation improves graft viability by reducing the release of pro-inflammatory molecules and the production of leukocyte adhesion molecules, thus increasing alveolar fluid clearance.(13,14)
The optimal timing for the administration of corticosteroids in the setting of brain death is controversial. The aim of the present study was to evaluate the effects that early and late systemic administration of methylprednisolone have on the lungs in a rat model of brain death.
MethodsThe Animal Care Committee and the Research Ethics Committee of the Porto Alegre Hospital de Clínicas, Federal University of Rio Grande do Sul, Porto Alegre, Brazil, approved the protocols used in the present study.
Twenty-four male Wistar rats, weighing 170-200 g, underwent general anesthesia, induced by intraperitoneal administration of ketamine (100 mg/kg) and xylazine (15 mg/kg). Anesthesia was followed by a tracheostomy with an indwelling 14-gauge cannula (Abbocath® #14; Abbott Laboratories, Abbott Park, IL, USA). The rats were ventilated with room air at 70-80 breaths/min with a tidal volume of 10 mL/kg (Harvard Rodent Ventilator, model 683; Harvard Apparatus Co., Millis, MA, USA). The right carotid artery was dissected and cannulated with a 24-gauge cannula (Becton Dickinson, Franklin Lakes, NJ, USA) for mean arterial pressure and HR recordings on an ink-jet recorder (Sirecust 730; Siemens-Elema, Solna, Sweden). The right jugular vein was dissected and cannulated in the same fashion. Normal saline was used to flush the lines, with a total volume of 5 mL/kg per hour in all animals, and a heated surgical table was used to maintain the body temperature at 37°C during the procedure.
After the initial procedures, the animals were randomly allocated to one of the following four groups (6 animals per group):
Sham (sham-operated): a craniotomy was performed, but no balloon catheter was introduced into the extradural space.
BD (brain death only): brain death was induced; at 5 min after brain death had been confirmed, 0.2 mL of normal saline was administered intravenously.
MP5 (methylprednisolone after 5 min of brain death): brain death was induced; at 5 min after brain death had been confirmed, an intravenous bolus of methylprednisolone (30 mg/kg) diluted in 0.2 mL of normal saline was administered .
MP60 (methylprednisolone after 60 min of brain death): brain death was induced; at 60 min after brain death had been confirmed, an intravenous bolus of methylprednisolone (30 mg/kg) diluted in 0.2 mL of normal saline was administered.
The brain death model has previously been described in detail.(15,16) In brief, through a frontolateral trepanation (1 × 1 mm with a dental drill), a 14G Fogarty balloon catheter (Baxter Healthcare Corp., Irvine, CA, USA) was introduced into the extradural space with the tip pointed caudally. The balloon was inflated with 1.5 mL of water for 1 min, producing a sudden rise in intracranial pressure, which resulted in rapidly progressive brain injury, leading to immediate brain death. A sharp rise and then a subsequent drop of blood pressure and HR defined the initiation of brain death. The state of brain death was confirmed by the absence of corneal reflexes and by the apnea test.
The animals were monitored for 120 min, during which the pre-procedure ventilation parameters were maintained. Arterial blood samples were drawn for blood gas analyses: at the time of the insertion of the arterial line (baseline); and at 60 and 120 min after the procedures. Upon completion of the assessment, a median sternotomy was performed, and the animals were sacrificed by exsanguination. The heart-lung blocks were excised, the right main bronchus was clamped, and the left lung was submitted to BAL three times (3 mL of normal saline each time). The right lung was then separated from the heart-lung block and divided into two parts. Most of the right lung was snap frozen in liquid nitrogen and stored at −80°C for subsequent analyses of lipid peroxidation, catalase activity, superoxide dismutase activity, and TNF- levels. The remainder of the lung was fixed in formalin for histopathology. The mediastinal lobe was excised and weighed (wet weight) on a precision scale. Samples were then placed in a vacuum oven (at 70°C for 72 h) until a stable dry weight had been achieved. The wet-to-dry ratio of the lung weight was then calculated and used as an indicator of lung edema.(17)
The BAL fluid (BALF) was centrifuged at 300 × g for 5 min, and the resulting cell pellet was separated from the supernatant. After preparation, the differential white cell count was determined with Romanowsky staining. The total protein concentration was determined using the biuret method (Modular Analytics; Roche Diagnostics, Penzberg, Germany), whereas the lactate dehydrogenase concentration was determined using the UV kinetic method (Modular Analytics).
After the samples had thawed, a 96-well plate was coated with monoclonal antibody for the determination of TNF- levels. The wells were filled with either 100 µL of homogenized lung (dilution 1:2), 100 µL of positive control, 100 µL of negative control, or 100 µL of recombinant TNF-, in concentrations established by the manufacturer of the antibody (Creative Biomart, New York, NY, USA). To each well, we then added 100 µL of polyclonal anti-TNF- conjugated with peroxidase and incubated the plates for 3 h at room temperature. After the incubation period, the plate was washed four times with a detergent solution. A color change was then induced by adding hydrogen peroxide (0.02%) and tetramethylbenzene (2%). The reaction was interrupted 30 min later with sulfuric acid (1 M). Color intensity was measured in an automated ELISA reader (Titertek Multiskan®; Flow Laboratories, McLean, VA, USA), at a wavelength of 450 nm, and is expressed as optical density. The TNF- concentration in the homogenized lung was calculated from the results obtained with a standard curve.
The products generated by lipid peroxidation were quantified by the thiobarbituric acid reactive substance (TBARS) method using 3 mg of protein per sample. The samples were incubated at 90°C for 30 min, and 500 µL of 0.37% thiobarbituric acid and 15% trichloroacetic acid were then added to the samples, which were centrifuged at 2,000 x g at 4°C for 15 min. Absorbance was determined using spectrophotometry at 535 nm.(18)
The activity of superoxide dismutase was determined by pulse radiolysis based on the auto-oxidation of epinephrine in accordance with the method described by Misra & Fridovich.(19) Catalase activity was determined by the spectrophotometry method described by Maehly & Chance.(20)
Myeloperoxidase activity was used as an indicator of polymorphonuclear leukocyte accumulation. The rate of change in absorbance was measured by spectrophotometry at 650 nm. Myeloperoxidase activity was defined as the quantity of enzyme degrading 1 µmol of peroxide/min at 37°C and was expressed in mU/g of wet tissue.
The fragment of lung fixed in formalin was embedded in paraffin, cut into 3-mm sections and stained with H&E. A pathologist blinded to the experimental protocol performed the quantitative examination by light microscopy. Each lung sample was examined at low and high magnifications, and 20 fields were randomly selected and analyzed. The severity of histological lesions was assessed using a five-parameter score that included intra-alveolar edema; hyaline membrane formation; hemorrhage; focal alveolar collapse or consolidation; and epithelial desquamation or necrosis of airways or alveoli. Each parameter was evaluated semi-quantitatively using the following scale: 0 = absent; 1 = mild; 2 = moderate; and 3 = prominent. For each animal, the parameter scores were summed in order to obtain the final score.(21)
All of the data collected were coded, recorded, and analyzed using the Statistical Package for the Social Sciences, version 16.0 (SPSS Inc., Chicago, IL, USA). We used ANOVA for comparisons among the groups. When ANOVA showed a significant difference, Tukey's post hoc test for multiple comparisons was applied in order to evaluate that difference. We used repeated measures ANOVA for intergroup comparisons related to dependent variables that were measured more than once during the observation period. For each test, the data were expressed as means and standard errors, and the level of significance was set at p < 0.05.
ResultsThere were no differences among the groups regarding the duration of the procedure; wet/dry weight ratio; arterial blood gas measurements; myeloperoxidase activity; superoxide dismutase activity; catalase activity; or (in the BALF) differential white cell count, total protein content, and lactate dehydrogenase levels. Nor were there any differences among the groups in terms of mean arterial pressure during the observation period.
The levels of TBARS were significantly higher in the two groups treated with methylprednisolone than in the sham and BD groups (p < 0.001; Figure 1). There were no significant differences in the histological scores among the groups (Figure 2). Lung injury, represented by intra-alveolar hemorrhage or hyaline membrane formation, was not observed in any samples. The predominant finding was mild focal alveolar collapse, which was present in the majority of the samples, at similar intensity across the groups (Figure 2). The levels of TNF- were significantly lower in the MP5 and MP60 groups when compared with the BD group (p < 0.001 for both; Figure 3).
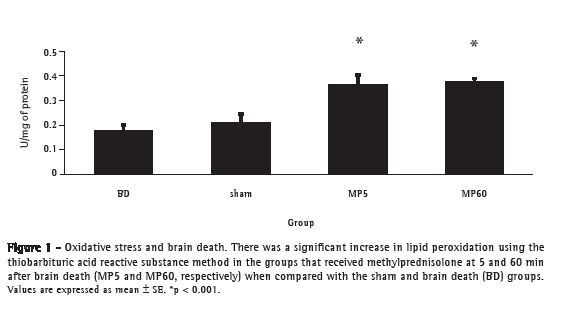
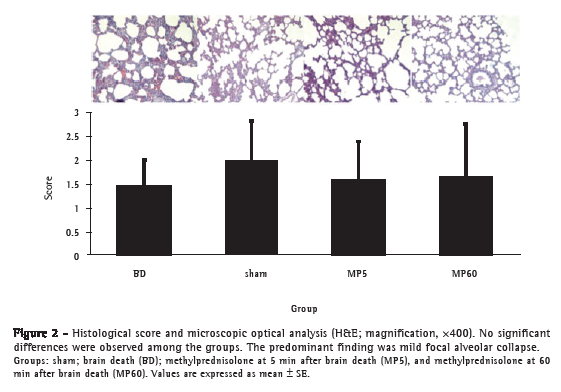
DiscussionThe vast majority of experimental studies have shown a significant increase in inflammatory molecules and interleukins immediately after the induction of brain death.(22,23) The pro-inflammatory cytokine TNF- has been shown to be significantly up-regulated in the lung tissue after brain death(12,24) and plays an important role in the development of lung injury.(22) In the present study, brain death resulted in a systemic inflammatory response and the administration of methylprednisolone was associated with reduced TNF- expression in lung tissue. McLean et al. obtained similar results in a study of the effects that a glucocorticoid has on myocardial function in a porcine model of brain death.(25)
To our knowledge, the present study is the first to investigate the role of the administration of methylprednisolone in a rat model of brain death and its influence on TNF- expression and oxidative stress in the lungs.(22)
Our study demonstrated that the administration of methylprednisolone has no effect on arterial blood gas and hemodynamic parameters. The lack of differences in these findings might be related to the short period of observation. The administration of methylprednisolone immediately after brain death in a rat model of lung transplantation has been shown to modulate the inflammatory injury of the donor lung, resulting in better graft performance after transplantation.(14)
Clinically, the use of corticosteroids in multi-organ donors is still controversial. However, various clinical studies using different hormone resuscitation protocols have shown promising results. Nevertheless, a standard protocol has yet to be established, especially with respect to lung retrieval.(26-29) The use of glucocorticoids in brain-dead donors probably has dual beneficial effects, because it reduces inflammatory activity and replaces cortisol levels in the blood. Although we studied TNF- activity after brain death, it would have been interesting to have also measured the cortisol levels after brain death in this model. In a baboon model, Faropoulos & Apostolakis found that cortisol levels rise within 5 min after the induction of brain death, declining steadily thereafter (over the next 15-45 min) and becoming undetectable at 4 h after brain death.(30)
In our experiments, we found no differences in the differential white cell count in BALF or in histology. We suppose that lung inflammatory changes can be assessed up to 120 min of ventilation after brain death induction in lungs that are still suitable for transplantation. However, there have been reports based on other experimental models of lung injury and brain death that advocate for the analysis of pulmonary inflammation within the first 6 h after brain death.(11)
The present study has limitations, and care must be taken when extrapolating its results to a clinical situation. We used a standardized, small animal model of brain death that includes a sudden rise in intracranial pressure, which differs from what is often found in patients evolving to brain death, in whom intracranial pressure rises at a slower pace. Another difference is that the time from brain death to organ harvest is typically much longer in the clinical scenario than it was in our model. A brain death model in which intracranial pressure is gradually induced by the inflation of a subdural balloon catheter has been developed. This improved model allows for the study of donor organ quality without the use of an inotropic infusion. Regarding corticosteroid administration, there is evidence that methylprednisolone given every 24 h (at 15 mg/kg i.v.) during brain death improves the effectiveness of the subsequent lung transplantation.(13) Therefore, we decided to use a higher dose of methylprednisolone in an attempt to potentiate the anti-inflammatory effects. The ideal period for the administration of the corticosteroid is still controversial in the literature. In the clinical scenario, it is expected that treatment with corticosteroids will be introduced as soon as possible in order to reduce the inflammatory activity. We considered 60 min after brain death to be late administration, given that, in a controlled animal model of brain death, the inflammatory activity at 60 min after brain death would be considerably higher than at 5 min after brain death.
The results of the present study show that the early administration of methylprednisolone after acute brain death does not improve oxygenation indices or hemodynamic parameters in lungs ventilated for 120 min. We also demonstrated an increase in the generation of lipid peroxidation products in the groups treated with methylprednisolone after 5 and 60 min of brain death. As previously demonstrated, traumatic brain injury significantly increases lipid peroxidation levels in lung tissue only 24 h after the trauma,(22) which could explain our lipid peroxidation results. However, the exact causes of these findings cannot be determined from our experiments.
We conclude that, in this model of brain death, early and late administration of methylprednisolone have similar effects on inflammatory and lipid peroxidation activity in lung tissue. We suggest that late administration of methylprednisolone can be used in settings of brain death, because of its potential anti-inflammatory effect. Further studies investigating the effects of late administration of methylprednisolone in the setting of lung transplantation following brain death are needed in order to confirm its beneficial effects.
References1. Trulock EP, Christie JD, Edwards LB, Boucek MM, Aurora P, Taylor DO, et al. Registry of the International Society for Heart and Lung Transplantation: twenty-fourth official adult lung and heart-lung transplantation report-2007. J Heart Lung Transplant. 2007;26(8):782-95.
2. Fernandes PM, Samano MN, Junqueira JJ, Waisberg DR, Noleto GS, Jatene FB. Lung donor profile in the State of São Paulo, Brazil, in 2006. J Bras Pneumol. 2008;34(7):497-505.
3. Organ and tissue donation and transplantation (update 2000). Canadian Medical Association [Article in English, French]. CMAJ. 2000;163(2):206-11.
4. Chakinala MM, Kollef MH, Trulock EP. Critical Care Aspects of Lung Transplant Patients. J Intensive Care Med. 2002;17:8-33.
5. Yeung JC, Cypel M, Waddell TK, van Raemdonck D, Keshavjee S. Update on donor assessment, resuscitation, and acceptance criteria, including novel techniques--non-heart-beating donor lung retrieval and ex vivo donor lung perfusion. Thorac Surg Clin. 2009;19(2):261-74.
6. Cypel M, Yeung JC, Liu M, Anraku M, Chen F, Karolak W, et al. Normothermic ex vivo lung perfusion in clinical lung transplantation. N Engl J Med. 2011;364(15):1431-40.
7. Avlonitis VS, Fisher AJ, Kirby JA, Dark JH. Pulmonary transplantation:
the role of brain death in donor lung injury. Transplantation. 2003;75(12):1928-33.
8. de Perrot M, Liu M, Waddell TK, Keshavjee S. Ischemia-reperfusion-induced lung injury. Am J Respir Crit Care Med. 2003;167(4):490-511.
9. Power BM, Van Heerden PV. The physiological changes associated with brain death--current concepts and implications for treatment of the brain dead organ donor. Anaesth Intensive Care. 1995;23(1):26-36.
10. Rosenberg RN. Consciousness, coma, and brain death--2009. JAMA. 2009;301(11):1172-4.
11. de Perrot M, Bonser RS, Dark J, Kelly RF, McGiffin D, Menza R, et al. Report of the ISHLT Working Group on Primary Lung Graft Dysfunction part III: donor-related risk factors and markers. J Heart Lung Transplant. 2005;24(10):1460-7.
12. Pratschke J, Neuhaus P, Tullius SG. What can be learned from brain-death models? Transpl Int. 2005;18(1):15-21.
13. Follette DM, Rudich SM, Babcock WD. Improved oxygenation and increased lung donor recovery with high-dose steroid administration after brain death. J Heart Lung Transplant. 1998;17(4):423-9.
14. Wigfield C, Golledge H, Shenton B, Kirby J, Dark J. Ameliorated reperfusion injury in lung transplantation after reduction of brain death induced inflammatory graft damage in the donor. J Heart Lung Transplant. 2002;21(1):57.
15. van Der Hoeven JA, Ter Horst GJ, Molema G, de Vos P, Girbes AR, Postema F, et al. Effects of brain death and hemodynamic status on function and immunologic activation of the potential donor liver in the rat. Ann Surg. 2000;232(6):804-13.
16. Herijgers P, Leunens V, Tjandra-Maga TB, Mubagwa K, Flameng W. Changes in organ perfusion after brain death in the rat and its relation to circulating catecholamines. Transplantation. 1996;62(3):330-5.
17. Pearce ML, Yamashita J, Beazell J. Measurement Of Pulmonary Edema. Circ Res. 1965;16:482-8.
18. Buege JA, Aust SD. Microsomal lipid peroxidation. Methods Enzymol. 1978;52:302-10.
19. Misra HP, Fridovich I. The role of superoxide anion in the autoxidation of epinephrine and a simple assay for superoxide dismutase. J Biol Chem. 1972;247(10):3170-5.
20. Maehly AC, Chance B. The assay of catalases and peroxidases: methods of biochemical analysis. In: Glick D, editor. Methods of Biochemical Analysis. New York: Interscience Publishers; 1954. p. 357-424.
21. Fujino Y, Goddon S, Chiche JD, Hromi J, Kacmarek RM. Partial liquid ventilation ventilates better than gas ventilation. Am J Respir Crit Care Med. 2000;162(2 Pt 1):650-7.
22. Barklin A. Systemic inflammation in the brain-dead organ donor. Acta Anaesthesiol Scand. 2009;53(4):425-35.
23. Takada M, Nadeau KC, Hancock WW, Mackenzie HS, Shaw GD, Waaga AM, et al. Effects of explosive brain death on cytokine activation of peripheral organs in the rat. Transplantation. 1998;65(12):1533-42.
24. Skrabal CA, Thompson LO, Potapov EV, Southard RE, Joyce DL, Youker KA, et al. Organ-specific regulation of pro-inflammatory molecules in heart, lung, and kidney following brain death. J Surg Res. 2005;123(1):118-25.
25. McLean KM, Duffy JY, Pandalai PK, Lyons JM, Bulcao CF, Wagner CJ, et al. Glucocorticoids alter the balance between pro- and anti-inflammatory mediators in the myocardium in a porcine model of brain death. J Heart Lung Transplant. 2007;26(1):78-84.
26. Van Bakel AB, Pitzer S, Drake P, Kay NA, Stroud M, Sade RM. Early hormonal therapy stabilizes hemodynamics during donor procurement. Transplant Proc. 2004;36(9):2573-8.
27. Venkateswaran RV, Patchell VB, Wilson IC, Mascaro JG, Thompson RD, Quinn DW, et al. Early donor management increases the retrieval rate of lungs for transplantation. Ann Thorac Surg. 2008;85(1):278-86; discussion 286.
28. Rosendale JD, Kauffman HM, McBride MA, Chabalewski FL, Zaroff JG, Garrity ER, et al. Aggressive pharmacologic donor management results in more transplanted organs. Transplantation. 2003;75(4):482-7.
29. Kutsogiannis DJ, Pagliarello G, Doig C, Ross H, Shemie SD. Medical management to optimize donor organ potential: review of the literature. Can J Anaesth. 2006;53(8):820-30.
30. Faropoulos K, Apostolakis E. Brain death and its influence on the lungs of the donor: how is it prevented? Transplant Proc. 2009;41(10):4114-9.
*Study carried out at the Porto Alegre Hospital de Clínicas, Federal University of Rio Grande do Sul, Porto Alegre, Brazil.
Correspondence to: Cristiano Feijó Andrade. Hospital de Clínicas de Porto Alegre, Departamento de Cirurgia Torácica, Rua Ramiro Barcelos, 2350, Santa Cecília, CEP 90035-903, Porto Alegre, RS, Brasil.
Tel. 55 51 3359-8839. E-mail: cristianofa@gmail.com
Financial support: This study received financial support from the Fundo de Incentivo à Pesquisa (FIPE, Research Incentive Fund) of Porto Alegre Hospital de Clínicas and Conselho Nacional de Desenvolvimento Científico e Tecnológico (CNPq, National Council for Scientific and Technological Development).
Submitted: 18 September 2012. Accepted, after review: 22 January 2013.
**A versão completa em português deste artigo está disponível em www.jornaldepneumologia.com.br
About the authorsEduardo Sperb Pilla
Thoracic Surgeon. Federal University of Rio Grande do Sul, Porto Alegre, Brazil.
Raôni Bins Pereira
Physician. Department of Thoracic Surgery, Porto Alegre Hospital de Clínicas, Federal University of Rio Grande do Sul, Porto Alegre, Brazil.
Luiz Alberto Forgiarini Junior
Professor of Physiotherapy. Methodist University Center, Porto Alegre Institute, Porto Alegre, Brazil.
Luiz Felipe Forgiarini
Biologist. Department of Thoracic Surgery, Porto Alegre Hospital de Clínicas, Federal University of Rio Grande do Sul, Porto Alegre, Brazil.
Artur de Oliveira Paludo
Medical Student. Federal University of Rio Grande do Sul School of Medicine, Porto Alegre, Brazil.
Jane Maria Ulbrich Kulczynski
Adjunct Professor. Federal University of Rio Grande do Sul School of Medicine, Porto Alegre, Brazil.
Paulo Francisco Guerreiro Cardoso
Professor. Department of Cardiorespiratory Diseases, University of São Paulo School of Medicine Hospital das Clínicas Heart Institute, São Paulo, Brazil.
Cristiano Feijó Andrade
Thoracic Surgeon. Porto Alegre Hospital de Clínicas, Federal University of Rio Grande do Sul, and Santo Antônio Children's Hospital, Santa Casa Hospital Complex, Porto Alegre, Brazil.