ABSTRACT
Objective: To describe the development of a silicone stent and perform in vivo testing for biocompatibility/applicability in the normal canine
trachea. Methods: Four different densities were tested in order to obtain the silicone prototypes. The pressure required for compression
considering a contact area of 1 cm2, and a 30% reduction in diameter was calculated for each density. The best density was 70-75 Shore
A hardness. Powdered barium sulfate was added to the silicone to make the stent radiopaque and easily identifiable in radiological
imaging. This novel stent presents a corrugated external surface with discontinuous and protruding arcs resembling the tracheobronchial
rings (for intercalation and fixation in the lumen of the lower airways), a highly polished inner surface and smooth extremities (to prevent
friction-related damage). The prototype considered most appropriate in terms of rigidity and flexibility was bronchoscopically implanted
in normal canine tracheas. After eight weeks, the animals were euthanized, and the tracheas were removed for anatomopathological
analysis. Results: There were no postimplantation complications, and none had to be removed. After eight weeks, the devices were found
to be well-positioned. Histopathology revealed a well-preserved epithelial basal membrane, foci of denuded epithelium, mild submucosal
inflammatory infiltrate with scattered granulation tissue, vascular neoformation, and no microorganisms. Conclusions: The stent developed
proved resistant to mechanical stress, biocompatible in the canine trachea and well-preserved at the study endpoint.
Keywords:
Implants, experimental; Silicones; Biocompatible materials.
RESUMO
Objetivo: Descrever o desenvolvimento de uma órtese de silicone e os testes in vivo de compatibilidade e aplicabilidade na traquéia canina
normal. Métodos: Quatro densidades de silicone foram testadas para a obtenção de protótipos. Para cada densidade, foi calculada a pressão
exigida para causar a compressão, considerando-se uma área de contato de 1 cm2 e uma redução de 30% no diâmetro. A densidade selecionada
foi 70-75 Shore A hardness. Adicionou-se sulfato de bário em pó ao silicone para tornar a órtese desenvolvida radiopaca e facilmente
identificável ao exame radiológico. A órtese desenvolvida apresenta superfície externa corrugada com arcos salientes e descontínuos, semelhantes
aos anéis traqueobrônquicos, para intercalação e fixação nas vias aéreas inferiores, superfície interna polida e extremidades lisas
que evitam o dano por fricção. O protótipo considerado como sendo o mais adequado em termos de rigidez e flexibilidade foi implantado
broncoscopicamente em traquéias caninas normais. Os animais foram sacrificados após oito semanas, e a traquéia foi removida para análise
anatomopatológica. Resultados: Não houve complicações após a implantação das órteses. Nenhuma precisou ser removida, e todas estavam
bem posicionadas ao final de oito semanas. O estudo histopatológico mostrou que a membrana basal epitelial foi preservada. Foram observados
pontos focais de desnudamento epitelial, leve infiltrado inflamatório sob a mucosa e, mais raramente, tecido de granulação com
neoformação vascular e ausência de microorganismos. Conclusões: A órtese desenvolvida mostrou resistência aos esforços mecânicos e
biocompatibilidade, não provocando reação tecidual adversa na traquéia canina, além de permanecer íntegra ao final do experimento.
Palavras-chave:
Implantes experimentais; Silicones; Materiais biocompatíveis.
IntroductionThe treatment of benign or malignant obstructive lesions of the lower airways has always been a challenge due to their complex pathophysiology and the various management options. Surgical correction is still the treatment of choice for most benign stenoses, especially those of medium size (1-6 cm in length) in the absence of an acute inflammatory process.(1,2) Nevertheless, surgery is contra-indicated in the presence of comorbidities such as chronic obstructive pulmonary disease, heart disease, diabetes and other systemic disorders, as well as after restenosis from previous surgery. In all such cases, the placement of stents has become a reliable alternative.(3,4)
Currently, there are more than 100 models of stent, which are available in different sizes, shapes and materials.(5) It has also become clear that there is no one ideal stent type for all tracheal applications.(6,7) Rather, the best option is largely determined by the anatomy of the lesion and of the airway.(8) Concerning materials, self-expanding metal stents and silicone stents seem to be the most widely employed.(2,7)
We have recently described the development and in vivo testing of a Nitinol stent.(9) In the present paper, we report on the development of a silicone stent and in vivo testing of that stent for biocompatibility and applicability in the normal canine trachea.
MethodsStent designThe silicone stent described here has been designated the HCPA-1 stent and is a modified version of the Dumon model.(10) The purported improvements are as follows: an external surface covered with discontinuous protruding arcs resembling the tracheobronchial rings (for intercalation and fixation in the lumen of the lower airways); a highly polished inner surface that prevents adhesion of dense mucus, blood or other materials from the respiratory tree; smooth extremities to decrease friction-related damage and the 'battering-ram' effect that can result from the impact of stent movement during breathing and coughing (Figure 1).
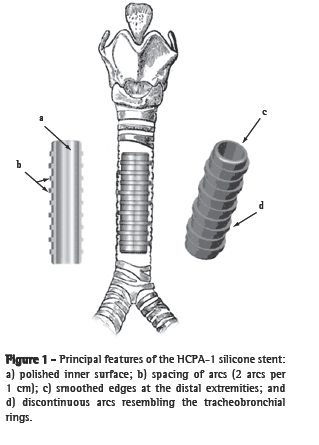
The HCPA-1 stent is made of biocompatible medical grade silicone through a process of injection molding (Pomp Medicone®, Cachoeirinha, Brazil) and has received patent number MU 7902500-5 from the Brazilian National Institute for Industrial Property.(11) Barium sulfate micropowder is added to the silicone to make the stent radiopaque and easily identifiable in radiological imaging studies.
In order to obtain data regarding a variety of characteristics related to rigidity and flexibility, prototypes of the silicone stent were prepared and tested at four different densities, expressed as the degree of Shore A hardness (SAH): 70-75 SAH; 25-28 SAH; 30-35 SAH; and 60-64 SAH. The size of the prototypes was defined based on radiographic images taken in profile from the cervicothoracic region.
A pusher device was built for introducing and placing the HCPA-1 stent into the tracheobronchial lumen. It is made of stainless steel and comprises a shaft, a hollow embolus that enables ventilation and a long metallic syringe with a beveled needle.
Mechanical assaysThe device employed for mechanical assays has been previously described.(9) In brief, tracheal stenosis is simulated to test each stent prototype in terms of its resistance to compression and displacement. The stents are placed horizontally on a scale (Al 500 model, Marte Balanças e Aparelhos de Precisão Ltda, São Paulo, Brazil) coupled to an LVDT T25 displacement sensor (Novotechnik, Stuttgart, Germany). Radial compression is gradually increased on the stent. The signals generated by the displacement sensors are acquired using an HBM Spider 8 data logger and the Catman Express® program, version 3.1. When the displacement sensor indicates a 30% reduction in stent diameter, the total mass recorded on the scale is read, and the weight force (or compression force) is calculated using the following formula:
F = ma
where force (F [Newtons, N]) is equal to the product of the mass (m [kg]) of the spheres multiplied by the acceleration of gravity (a [m/s2] ≈ 9.81 m/s2).
The pressure required for displacement was calculated for each density considering a contact area of 1 cm2: 70-75 SAH, 2.4 N/cm2; 25-28 SAH,
0.5 N/cm2; 30-35 SAH, 1.1 N/cm2; and 60-64 SAH, 1.75 N/cm2 (Figure 2).
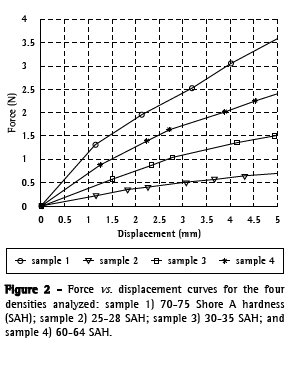
Sample 1 (70-75 SAH) tolerated the highest load with the least displacement and was therefore chosen for the animal experiments. The dimensions of the prototype used in the animal studies were as follows: length, 50 mm; external diameter (excluding arcs), of 10 mm; internal diameter, 8 mm; wall thickness, 1 mm; and external arcs, 0.5-1.5 mm.
Animal experimentsThe canine model was chosen based on the close similarity between the lower airways of dogs and those of humans in terms of format and dimensions. The experimental animals were obtained from the Veterinary School Hospital at the Federal University of Rio Grande do Sul (Porto Alegre, Brazil). Prior to the procedures, the animals selected were quarantined in the university kennel, where they were vaccinated and treated for parasitic infections.
They were subsequently submitted to a preliminary evaluation (clinical and radiological), after which they were monitored on a daily basis for changes in vital signs and signs of disorders. During all procedures, from surgery to sacrifice, the animals received humane care in terms of analgesia and sedation in compliance with well-established bioethical guidelines.(12) The study received approval from the Research Ethics Committees of the Hospital de Clínicas de Porto Alegre and the Veterinary School Hospital.
The sample included five one- to three-year-old mongrel dogs weighing 10-20 kg, without tracheobronchial anomalies. Anesthesia was performed after a fast (12 h without food and 3 h without water). The animals received 0.044 mg/kg of body weight (BW) subcutaneous atropine sulfate and 1 mg/kg BW intramuscular xylazine. After 15 min, general anesthesia was induced with 12.5 mg/kg BW intravenous sodium thiopental under spontaneous ventilation.
We used a rigid bronchoscope (10339 CC; Karl Storz, Tuttlingen, Germany) with a 26-cm stainless steel shaft (6 mm internal diameter; 6.7 mm external diameter) and a frontal telescope (2.9 mm in diameter; 30 cm in length), together with a prism light deflector, a suction catheter, biopsy forceps, a 2.3-m fiber optic cable, a cold light source with two halogen lamps (24 V/250 W), a 36-cm Trinitron color video monitor (Sony, San Diego, CA, USA) with a horizontal resolution of 525 TV lines, and an XL microcamera for endoscopy (Karl Storz).
The dog was placed on its back with its head extended, and the bronchoscope was introduced under direct viewing after exposure of the larynx, with the aid of a laryngoscope or by careful external traction of the tongue.
The stent was introduced into the pusher coupled to the bronchoscope.(11) The silicone stent remained folded longitudinally inside the embolus until placement. Placement was made after direct bronchoscopic visualization of the selected site. After the embolus is pushed, complete expansion of the stent is achieved with help of traction or rotation movements guided by the biopsy forceps. A suture was placed through the skin to ensure fixation of the stent on the tracheal surface. This was done to prevent displacement or expulsion of the stent, since the diameter of the normal canine trachea varies greatly in response to stimuli such as heat, exercise, stress or anesthesia.
The animals were evaluated every two weeks with bronchoscopic and radiographic revisions for evaluation of stent positioning. After eight weeks, the animals were euthanized under general anesthesia with 1 mL/kg BW endovenous magnesium sulfate infusion.
Following euthanasia, the tracheal stump containing the stent was removed for pathological examination. The macroscopic and microscopic analysis focused on the presence or absence of preserved mucosa, leukocyte infiltration, exudate, granulation, vascular neoformation, mucosal adherence, suppuration or perforations. A fine brush was used to collect the exudate covering the inner and outer stent surface for cell block preparations. Several cross sections of the central and lateral trachea were obtained, some from portions in contact with the stent and others from portions not in contact. The samples were fixed in formalin and stained with hematoxylin-eosin, Gram or Grocott's methenamine silver, after which they were evaluated under optical microscopy. Cytological analysis and the identification of pathogens were performed in the tracheal sections and in the cell block.
Results There were no remarkable postimplantation events or complications, and there were no cases in which the need to remove a stent arose. At eight weeks after implantation, the devices were found to be well-positioned (Figures 3 and 4). Histopathologic analysis of the tracheal specimens showed a well-preserved epithelial basal membrane. As shown in Figure 5, a small quantity of submucosal inflammatory infiltrate, consisting predominantly of polymorphonuclear cells, was frequently observed, together with sparse areas of granulation tissue and vascular neoformation. No microorganisms were detected.
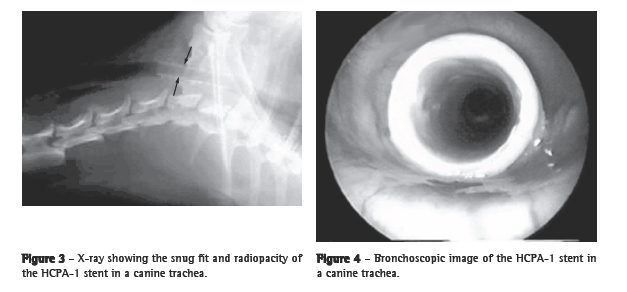
DiscussionThere were no technical difficulties encountered during the use of the HCPA-1 silicone stent. The external arcs successfully increased adherence at the implantation site. We believe that the proposed stent model would be ideal for applications involving the trachea and proximal bronchi. The mechanical assays were appropriate for determining the properties of silicone prototypes of various densities. These assays can be easily reproduced using the present methodology to evaluate various diameters, degrees of rigidity or radial strength for a broad range of applications.
A common complication associated with the normal canine trachea is the displacement and consequent migration of the stent, due to the lack of obstacles to mucosal adherence. This was resolved in our study by the placement of an external suture. However, it should be borne in mind that, in the presence of stenosis, migration is less likely to occur.(5,8) In humans, Colt has also described the use of external sutures during stent placement in the upper portion of the trachea, which is more mobile.(13) However, we believe that suturing would rarely be required for fixation of the HCPA-1 stent.
Among the disadvantages of silicone stents are the need for rigid bronchoscopy during placement, difficulties in deployment and internal diameter/ external diameter ratios that are less favorable than those of metal stents. In the present study, we attempted to optimize the relationship between stent wall thickness and radial strength by testing different densities in order to determine which would best tolerate the tracheal pressure on the stent walls.(2,3,6,14)
Other simplified silicone prototypes, such as the Tygon model, have been tested, with relative success.(15) Additional tests involving bioabsorbable stent materials that approach the ideal are in progress.(16) The model employed in the present study appears to have some advantages over earlier silicone stents. The absence of hooks or rough edges seems to ensure a more amenable adhesion to the mucosal surface. It is also of note that we did not observe stent-induced lesions such as rough epithelium denudation, pyogenic granulomas or even fistulae, all of which have been previous described.(2,5,6,14,17) We believe that this was possible due to the anatomical design and to the use of highly biocompatible silicone, which is appropriate for long-term implantation even in humans. In addition, silicone stents are still considered safer than metallic stents for benign disorders.(18) One study(19) employing the HCPA-1 stent is currently underway in patients with benign and malignant tracheobronchial stenosis.
In conclusion, the mechanical assays performed allowed us to develop a tracheal stent that behaved well in the chosen experimental animal model. The results of future studies will allow us to determine whether the model proposed is appropriate, in terms of density and design, for use in the human trachea and bronchi.
References1. Colt HG, Dumon JF. Airway stents. Present and future. Clin Chest Med. 1995;16(3):465-78.
2. Ernst t A, Feller-Kopman D, Becker HD, Mehta AC. Central airway obstruction. Am J Respir Crit Care Med. 2004;169(12):1278-97.
3. Ciccone AM, De Giacomo T, Venuta F, Ibrahim M, Diso D, Coloni GF, et al. Operative and non-operative treatment of benign subglottic laryngotracheal stenosis. Eur J Cardiothorac Surg. 2004;26(4):818-22.
4. Saueressig MG, Macedo Neto AV, Moreschi AH, Xavier RG, Sanches PR. A correção das estenoses traqueobrônquicas mediante o emprego de órteses. J Pneumol. 2002;28(2):84-93.
5. A survey of stent designs. Minim Invasive Ther Allied Technol. 2002;11(4):137-47.
6. Venuta F, Rendina EA, De Giacomo T, , Mercadante E, Ciccone AM, Aratari MT, et al. Endoscopic treatment of lung cancer invading the airway before induction chemotherapy and surgical resection. Eur J Cardiothorac Surg. 2001 Sep;20(3):464-7.
7. Wood DE, Liu YH, Vallières E, Karmy-Jones R, Mulligan MS. Airway stenting for malignant and benign tracheobronchial stenosis. Ann Thorac Surg. 2003;76(1):167-72; discussion 173-4.
8. Freitag L, Eicker K, Donovan n TJ, Dimov D. Mechanical properties of airway stents. J Bronchol. 1995;2(4): 270-8.
9. Vearick SB, Michelon MD, Schaeffer L, Xavier RG, Kuhl G, Sanches PR, et al. Development and in vivo testing of a Nitinol tracheal stent. J Biomed Mater Res B Appl Biomater. 2007;83(1):216-21.
10. Dumon n JF. A dedicated tracheobronchial stent. Chest. 1990;97(2):328-32.
11. Xavier RG, Sanches PR, Macedo Neto AV, Silva Filho AP, Edelweiss MI, Duarte L, et al. Report of a new silicone stent bronchoscope introducer system (HCPA-1) studied in dogs. Chest. 2000;118(Suppl 4):234S.
12. Smith PC, Taylor V, coordinators. Environmental enrichment information resources for laboratory animals: 1965-1995. Beltsville, MD: Animal Welfare Information Center of the US Agriculture Department, National Agriculture Library, Universities' Federation for Animal Welfare; 1995.
13. Colt HG. Silicone airway stents. In: Beamis Jr JF, Mathur PN, editors. Interventional pulmonology. New York: McGraw-Hill; 1999, p. 97-112.
14. Fraga a JC, Filler RM, Forte V, Bahoric A, Smith C. Experimental trial of balloon-expandable, metallic Palmaz stent in the trachea. Arch Otolaryngol Head Neck Surg. 1997;123(5):522-8.
15. Noppen M, Dhaese J, Meysman M, Monsieur I, Verhaeghe W, Vincken W. A new screw-thread tracheal endoprosthesis. J Bronchol. 1996; 3(1): 22-6.
16. Saito Y, , Minami K, Kobayashi M, Nakao Y, Omiya H, Imamura H, et al. New tubular bioabsorbable knitted airway stent: biocompatibility and mechanical strength. J Thorac Cardiovasc Surg. 2002;123(1):161-7.
17. Noppen M. Airway injury and sequelae: conservative view. Eur Respir Mon 2004;9(29): 234-45.
18. U.S. Food and Drug Administration - Center for Devices and Radiological Health [Homepage on the Internet]. Rockville MD: Center for Devices and Radiological Health/CDRH; FDA. [updated 2005 Aug 2; cited 2006 Jun 30]. Complications from metallic tracheal stents in patients with benign airway disorders; [about 4 screens]. Available from: http://www.fda. gov/cdrh/safety/072905-tracheal.html
19. Macedo Neto AV, , Gonçalves L, Xavier RG, Sanches PR, Saueressig M, Moreschi A, et al. Report on a new silicone stent for the human trachea and bronchi [abstract]. XIII World Congress for Bronchology; 2004 Jun 20-23; Barcelona, Spain: p. 100.
_________________________________________________________________________________________________________________
Study carried out at the Porto Alegre Hospital de Clínicas. Federal University of Rio Grande do Sul, Porto Alegre, Brazil.
1. Adjunct Professor in the Internal Medicine Department. Federal University of Rio Grande do Sul, Porto Alegre, Brazil.
2. Researcher at the Experimental Airway and Lung Laboratory. Porto Alegre Hospital de Clínicas Research Center, Porto Alegre, Brazil.
3. Adjunct Professor in the Thoracic Surgery Department. Porto Alegre Hospital de Clínicas, Porto Alegre, Brazil.
4. Assistant Professor in the Department of Otorhinolaryngology. Porto Alegre Hospital de Clínicas, Porto Alegre, Brazil.
5. Researcher at the Mechanical Transformation Laboratory. Federal University of Rio Grande do Sul, Porto Alegre, Brazil.
Correspondence to: Rogério Gastal Xavier. Avenida Soledade, 478, CEP 90470-340, Porto Alegre, RS, Brasil.
Tel 55 51 3388-5000. E-mail: rxavier@terra.com.br
Submitted: 11 January 2007. Accepted, after review: 10 May 2007.
.**A versão completa, em português, deste artigo está disponível em: www.jornaldepneumologia.com.br