ABSTRACT
Objective: To test the applicability of a geometric model, adapted to the supine position, for the analysis of respiratory mechanics regarding changes in lateral thoracoabdominal areas in children with asthma. Methods: Nineteen children (mean age, 11.26 ± 1.28 years) performed isovolume maneuvers (IVMs) after maximal inspiration, followed by glottal closure and alternation of airflow between the abdominal and thoracic compartments. The maneuvers were recorded in a digital video camera placed perpendicularly to the movement plane, and the images of interest were selected. The geometric model was traced on each image based on surface landmarks of anatomical references. The traced areas were calculated using a computer program, and the results were converted into metric units (cm2) using a surface landmark of a known area. Relative contributions (RCs) of the subcompartments in relation to their original compartments and to the chest wall (CW) were calculated. Results: The model was based on 55 thoracic IVM images and 55 abdominal IVM images. Areas and subareas were compared between the maneuvers. There were significant differences in all subcompartments (p < 0.001). All of the RCs were significantly different for the CW (p < 0.001) but not for the ratios between the subcompartments and their original compartments. Conclusions: This geometric model, applied in children and adapted to the supine position, was effective in profiling changes in the thoracoabdominal silhouette during the IVMs, and the selected subdivisions were useful for the identification of areas contributing the most and the least to CW composition.
Keywords:
Photogrammetry; Respiratory mechanics; Image processing, computer-assisted.
RESUMO
Objetivo: Testar a aplicabilidade de um modelo geométrico, adaptado à postura deitada, para a análise da mecânica respiratória em relação à variação das áreas toracoabdominais laterais em crianças asmáticas. Métodos: Dezenove crianças (média de idade: 11,26 ± 1,28 anos) realizaram manobras de isovolume (MIV) após inspiração máxima, seguida de fechamento da glote e alternância do ar entre os compartimentos abdominal e torácico. As manobras foram filmadas por uma câmera digital perpendicular ao plano de movimento, e as imagens de interesse foram selecionadas. O modelo geométrico foi traçado sobre cada imagem, orientado por marcadores de superfície em referências anatômicas. As áreas traçadas foram calculadas através de um programa, e os resultados foram convertidos
para unidades métricas (cm2) utilizando um marcador de superfície de área conhecida. Foram calculadas as contribuições relativas (CRs) dos subcompartimentos em relação ao seu compartimento de origem e à parede torácica (PT). Resultados: O modelo foi medido a partir de 55 imagens de MIV torácicas e de 55 de MIV abdominais,
e as áreas e subáreas entre as manobras foram comparadas, havendo diferenças significativas para todos os subcompartimentos (p < 0,001). Todas as CRs, em relação à PT, foram também significativamente diferentes (p < 0,001), mas não aquelas em relação às razões entre os subcompartimentos e os compartimentos de origem. Conclusões: Este modelo geométrico, aplicado em crianças e adaptado à postura deitada, foi efetivo na diferenciação
das variações do contorno toracoabdominal durante as MIV, e os níveis de divisão propostos foram úteis na identificação das regiões mais e menos contributivas à composição da PT.
Palavras-chave:
Fotogrametria; Mecânica respiratória; Processamento de imagem assistida por computador.
IntroductionThe isovolume maneuver (IVM),(1,2) or maneuver for determining isovolumetric variations in the thorax and abdomen, was created as a calibration method for surface analysis in the study of respiratory mechanics. The IVM is a relevant clinical expression of the relationship between the coupling forces of the rib cage and abdomen.(3) These forces result in controlled respiratory muscle activation(4) during lung volume change.
The IVM was first described in 1967 in a study analyzing the kinetic relationships between the thoracic and abdominal compartments at various volumes.(1) This maneuver corrects the relationships between the movements of the rib cage and those of the abdomen, creating a volume balance between the two, so that, during surface measurements, similar inflections in thoracic and abdominal signals indicate a similar, proportional contribution to the observed tidal volume.(5) The IVM is performed in order to determine the possible variations between the thoracic and abdominal components, under conditions that result in airway closure, such as simultaneous occlusion of the mouth and nose. Under such conditions, the respiratory system has only one degree of freedom of movement, which means that, at a given volume, any change in the movement of the rib cage is probably inversely proportional to that of the abdomen.(6)
Muscle activity while performing an IVM is not similar to the standard mechanism observed during respiration. On the contrary, inward displacement of the abdomen during thoracic expansion is a clinical sign of respiratory distress.(3) Therefore, systematization of the IVM for research purposes requires that volunteers learn how to perform the maneuver, through prior training or graphic feedback, before it can be used for measurements.(1)
The question refers to the mechanics involved in the conscious control of the thoracic and abdominal respiratory movement(7) and is directly related to the development of strategies for the care of chronic respiratory disease patients, especially children.(8,9) This maneuver is related to the conscious control of muscle activity using an intentional mechanical strategy. It is successfully executed when the diaphragm and intercostal muscles are alternately mobilized to induce a change in air distribution among the lung regions.(10)
This concept is fundamental to the development of new technologies that use the movement of the thoracoabdominal surface to measure variation in lung volume. The purpose of this study was to submit a geometric model of photogrammetry, designated biofotogrametria para análise da mecânica respiratória (BAMER, photogrammetric analysis of respiratory mechanics) and previously used in adults, to the analysis of variations in the thoracoabdominal contour during IVMs in children, after the inhalation of a given volume of air that approximates total lung capacity.
MethodsThe procedures used in the present study were approved by the Ethics Committee of the Hospital de Clínicas da Universidade Federal do Paraná (HC/UFPR, Federal University of Paraná Hospital de Clínicas), in accordance with Brazilian National Health Council Resolution 196/96. This was a descriptive, observational study that analyzed the IVMs performed by 19 children with asthma, aged 9-13 years, who had been treated for at least 12 months and were stable for at least 30 days prior to testing. The children were screened at the outpatient clinic of the Pediatric Allergy, Immunology and Pulmonology Section of the HC/UFPR in Curitiba, Brazil, and at a specialized unit in Paranaguá, Brazil, between October and December of 2006.
The children who participated in this study were previously instructed in how to perform the IVM and underwent routine respiratory muscle testing by means of nonspecific diagnostic tests, namely the determination of maximal respiratory pressure and PEF, as well as the measurement of thoracic and abdominal circumferences, during maximal respiratory maneuvers. All tests were performed while the patients were seated, and each test was repeated three times; these tests, however, are not the subject of discussion in the present study.
Image acquisitionBecause children typically cannot remain still while performing maximal respiratory maneuvers, we opted for performing the IVMs in the supine position, with lumbosacral rectification performed by the rater through manual stretching. The upper limbs were flexed, abducted and rotated outwards, with the hands placed under the cervical curvature, the elbows being completely abducted (Figure 1).
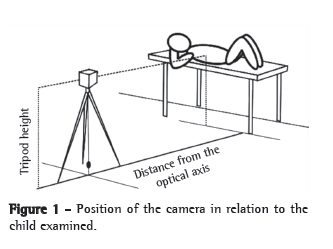
After the initial tests, flat spherical surface markers (13 mm in diameter) were placed at the following locations: (1) anterior superior iliac spine; (2) lateral to the umbilicus; (3) the lower angle of the 10th rib cartilage; (4) lateral to the xiphoid process; and (5) lateral to the jugular notch of the sternum. These points served as landmarks for the geometrical delineation of the thoracoabdominal compartments in the images acquired during the IVMs. The final tracing resulted in an adaptation of the BAMER model for the supine position.(11)
After a number of trial runs, images of the IVMs were acquired. To measure the volume of air inhaled prior to the maneuvers, the procedures were performed in the following sequence: (a) positioning of a nose clip and mouthpiece of an analogical spirometer (model RM-121; Ohmeda, Boulder, CO, USA), with a minimum sensitivity of 10 mL, the measurement feature of which was deactivated; (b) moderate inhalation, followed by active exhalation to near residual volume; (c) activation of the measurement feature of the spirometer, followed by a maximal inhalation to near total lung capacity; (d) post-inspiratory suspension of breathing, in order to read the volume measured and adjust the inhalation pattern; (e) removal of the mouthpiece followed by glottal closure to maintain the volume inhaled; (f) performance of the IVM, alternating between the Müller maneuver, or abdominal IVM (AIVM), and a maneuver of thoracic inhalation with abdominal exhalation, or thoracic IVM (TIVM). For each maneuver, abdominal and thoracic positions were maintained for 5 sec, and the entire process was performed three to five times.
Image processingOne film was generated for each child. From these films, we extracted images of the maneuvers at stable time points. Image extraction was performed using the Corel R.A.V.E.® computer program (Corel Corp., Ottawa, Ontario, Canada). Each photogram selected was exported to the CorelDraw® computer program, version 12 (Corel Corp.), which was used to trace the thoracoabdominal divisions based on the surface landmarks.
Construction of the adapted BAMER model was defined by irregular quadrilaterals, traced on the images from planes transverse to the chest, guided by the surface markers. The area of the chest wall (CW) was delineated superiorly at the level of the jugular notch of sternum and inferiorly at the level of the anterior superior iliac spine. This area was divided at the level of the xiphoid process into the thoracic compartment (TC) and the abdominal compartment (AC). Each compartment was then subdivided, forming a total of four subcompartments (Figure 2).
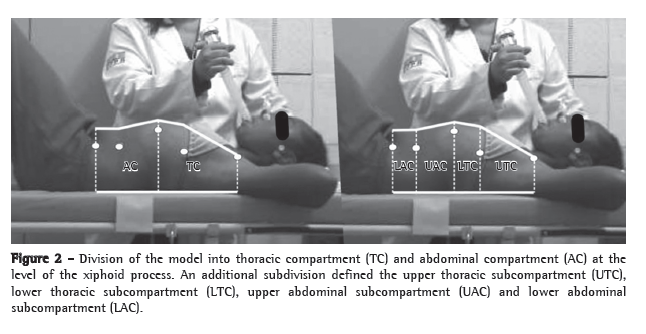
At the level of the xiphoid process, the TC was divided into upper and lower thoracic subcompartments. At the level of the lower angle of the 10th rib cartilage, the AC was divided into upper and lower abdominal subcompartments. The quadrilaterals were delineated by the upper and lower connection between the limits of the anterior and posterior thoracoabdominal contours.
The BAMER model tracing for each maneuver was then exported to the AutoCAD® 2005 program (Autodesk Inc., San Rafael, CA, USA), which was used to calculate the lateral areas, using the area of one of the surface markers (1.69 cm2) to calibrate the conversion. In the divisions of the model, the result of the mathematical combination of the lateral areas made it possible to calculate the partial and total relative contributions (RCs) of each subcompartment, in relation to its original compartment as well as to the CW as a whole.
Statistical analysis of the results was performed using the program Statistical Package for the Social Sciences, version 13.0 (SPSS Inc., Chicago, IL, USA), applying the Kolmogorov-Smirnov test to assess the normal distribution of the data, and paired parametric inferential tests to assess each level of model division, together with analysis of the differences between maneuvers in terms of the RCs. For all of the analyses, the level of significance was set at p < 0.05.
ResultsOf a total of 25 children screened, 22 agreed to participate in this study. At the end of the process of evaluation and filming, there were technical problems with the films for three children. Therefore, the results include data from only 19 children. Of those 19 children, 8 were female and 11 were male. The mean age was 11.26 ± 1.28 years. In 58% of the cases, the asthma was accompanied by a diagnosis of rhinitis. The BAMER model was applied to images of 110 maneuvers, of which 55 were TIVM images and 55 were AIVM images.
Comparison of results for the same compartments vs. maneuversTable 1 shows the descriptive statistical analysis and the percentile distribution of the results obtained for the measurements at each division of the model. The Kolmogorov-Smirnov test showed normal distribution of the data, which allowed the use of parametric inferential tests for the comparative analysis of the results.
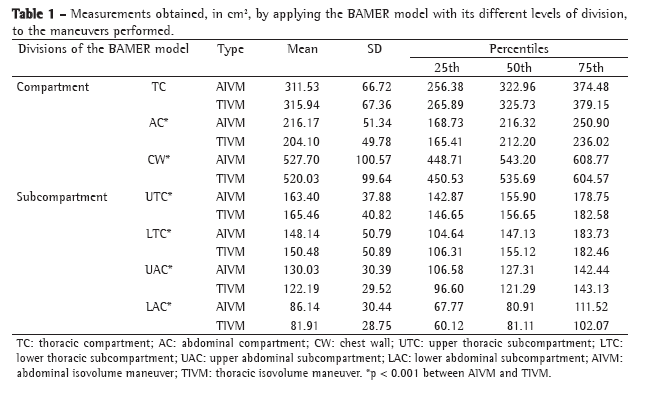
The Student's t-test was applied to compare AIVM and TIVM for each division of the BAMER model. The results showed significant differences between the divisions (p < 0.01).
Analysis of the relative contributions of the divisions of the modelThe RCs are dimensionless and were calculated based on the following ratios: (1) between the area of the division and the total estimated area of the CW; (2) between the area of the subcompartment and that of its original compartment. The results are shown in Table 2.
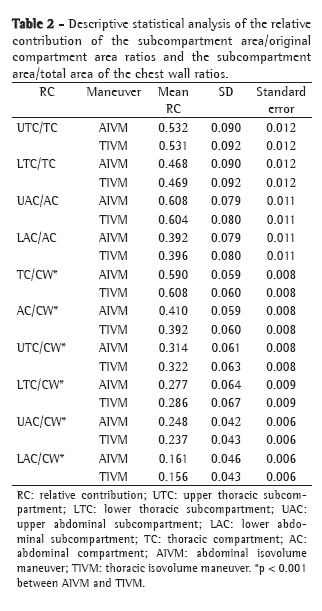
The comparative analysis of TIVM and AIVM using the Student's t-test showed that the means of the RC ratios regarding the CW were significantly different (p < 0.001). There was no difference in the RCs of the ratio of the subcompartments to their original compartments.
DiscussionIn general, quantitative measurements of respiratory movements are based on the measurement of lung volume and of the displacement of the thoracoabdominal structures caused by lung volume. Because the tissues of the CW are essentially incompressible, variations in lung volume result in thoracoabdominal displacement. Measurements of such displacement can be used for noninvasive volume estimates, without the need to use an interface, such as a mouthpiece or a mask.(4)
According to the two-compartment model proposed by Konno and Mead,(1) the rib cage and abdomen move independently during breathing at rest in most people. Simultaneous records of the thoracic and abdominal displacements are necessary to precisely measure thoracoabdominal displacement that is directly related to the variation in lung volume.(4) The activation of the intercostal muscles and accessory inspiratory muscles causes thoracic expansion, promoting a counter abdominal retraction. In contrast, activation of the abdominal muscles contracts the abdomen,
causing abdominal expansion, via an increase in abdominal pressure, as well as thoracic retraction, via a direct effect of the insertions of these muscles at the costal margin.(12)
In studies of respiratory mechanics based on thoracoabdominal displacement, the CW is considered a linear elastic system with two degrees of freedom of movement.(13) In this context, the diaphragm has different effects on the TC and AC. During breathing at rest, the diaphragm causes outward displacement of the entire CW, whereas, during the Müller maneuver, the abdominal wall is displaced outwards at the same time that the rib cage is contracted. Upon glottal closure, this system has only one degree of freedom of movement, and the gain between thoracic and abdominal signals is similar. Thus, it is possible to change the thoracoabdominal contour, causing the alternate displacement of the volume of one compartment to the other, i.e., alternating between the performance of the Müller maneuver and the contraction of the intercostal and accessory inspiratory muscles.(14,15)
The first studies of isovolume pressure-flow curves date back to the 1960s.(16,17) They showed that the value for stabilization of the respiratory system, upon closure of the airways, was determined solely by lung volume. Since achieving balance of the flow did not depend on effort, the singular relationship between maximum flow rate and lung volume was easily determined by a graph including the variables flow rate and lung volume, obtained during a single exhalation, at FVC. Thus, the maximal expiratory volume-flow curve was created as the third plane of a 3D graph
of the respiratory mechanics.(2)
Konno & Mead systematized and introduced the IVM as a resource for the calibration of instruments to measure variations in lung volume by determining thoracoabdominal displacement.(1) This strategy has been used by most researchers working in the field of respiratory mechanics.(15) The thoracoabdominal contour and movement can be determined using various surface methods, according to various physical principles, such as magnetometry,(18) inductive plethysmography,(19) kinetic imaging(20) and light projection.(21) Two- or three-dimensional information obtained with any of these methods is used to infer clinically relevant aspects of the respiratory mechanics.
The use of photogrammetry for the analysis of the two-dimensional thoracoabdominal respiratory movement has made it possible to obtain, in a clear and simple manner, evidence regarding relationships between movement and volume under known conditions, as well as results previously described using more robust systems.(13,15) This is possible because the BAMER model is able to quantify the differences between the AIVM and TIVM in terms of the accommodation of the areas.(10) The use of the supine position to test the BAMER model in children in the present study is in accordance with the corollary stating that a fixed position is required for the IVMs to be executed.(13)
In view of the results obtained, we can propose the application of the BAMER model to observe the evolution of respiratory muscle control resulting from strategies such as respiratory therapy,(22,23) global postural reeducation(24) and Pilates training, used as complements to the standard treatment of respiratory disease. These complementary resources make use of the determination of IVM variations as a technique for respiratory exercises, in which the control of the maneuver is conscious and voluntary. It is fundamental that the active and passive forces acting upon the CW during the respiratory movement be controlled by muscular, elastic and pressure elements, so that a person performing an IVM has voluntary control of all these elements. The BAMER model has proven effective at taking measurements in this context.
The levels of division proposed in the BAMER model were used to identify relevant aspects while monitoring patients recovering from primary or secondary respiratory disease(25) and performing an abdominal maneuver. This was done when assessing the magnitude of voluntary control of the respiratory muscles, as in respiratory rehabilitation exercises.(22) The techniques used in respiratory exercises are among the most popular complementary medical techniques, being employed by patients with asthma or other respiratory diseases. Respiratory exercises, such as yoga and diaphragm respiration, lead to a reduction in the use of short-acting β2 agonists and to an improvement in the quality of life.(26)
Although it is imperative that further studies involving the use of photogrammetry in different clinical situations and in different age groups be conducted, the BAMER model represents a promising resource for the quantitative follow-up evaluation of patients. The quantification of the intensity of the performance of the IVM and the manner it is performed, through measurement of the RC rates of each compartment, are relevant contributions of the BAMER model. It can also be used in order to give daily feedback in respiratory rehabilitation programs, as well as to provide therapeutic motivation to respiratory disease patients, especially children and the elderly. The limitations of the method should be further investigated so that it can be used, in the future, as a complementary resource in the physical and functional examination of respiratory muscle activity.
We conclude that the BAMER model applied in children and adapted to the supine position was effective in profiling variations in the thoracoabdominal contour during the performance of IVMs. The subdivisions of the model allowed us to identify more accurately the RC of each division to the total variation of the CW. These findings indicate the need for further studies exploring the use of this model for analyses prior to and after the implementation of therapeutic strategies that might improve voluntary control of the respiratory muscles, as well as for the analysis of the performance of therapeutic and diagnostic maneuvers, especially in children.
AcknowledgmentsThe authors would like to thank the Grupo de Apoio ao Programa de Educação Respiratória (GAPER, Support Group of the Respiratory Education Program) of the city of Paranaguá, Brazil, for allowing us conduct the present study in their facilities.
References 1. Konno K, Mead J. Measurement of the separate volume changes of rib cage and abdomen during breathing. J Appl Physiol. 1967;22(3):407-22.
2. Macklem PT. The mechanics of breathing. Am J Respir Crit Care Med. 1998;157(4 Pt 2):88-94.
3. Prisk GK, Hammer J, Newth CJ. Techniques for measurement of thoracoabdominal asynchrony. Pediatr Pulmonol. 2002;34(6):462-72.
4. American Thoracic Society/European Respiratory Society. ATS/ERS Statement on respiratory muscle testing. Am J Respir Crit Care Med. 2002;166(4):518-624.
5. Gilbert R, Auchincloss JH Jr, Peppi D. Relationship of rib cage and abdomen motion to diaphragm function during quiet breathing. Chest. 1981;80(5):607-12.
6. Sackner MA, Watson H, Belsito AS, Feinerman D, Suarez M, Gonzalez G, et al. Calibration of respiratory inductive plethysmograph during natural breathing. J Appl Physiol. 1989;66(1):410-20.
7. Laghi F, Tobin MJ. Disorders of the respiratory muscles. Am J Respir Crit Care Med. 2003;168(1):10-48.
8. Luce JM, Culver BH. Respiratory muscle function in health and disease. Chest. 1982;81(1):82-90.
9. Postiaux G. Fisioterapia Respiratória Pediátrica: O tratamento guiado por ausculta pulmonar. Porto Alegre: Artmed; 2004.
10. De Groote A, Paiva M, Verbandt Y. Mathematical assessment of qualitative diagnostic calibration for respiratory inductive plethysmography. J Appl Physiol. 2001;90(3):1025-30.
11. Ricieri DV, Rosário-Filho NA. Assessing the impact that external factors have on respiratory mechanics assessed using a specific photogrammetric model. J Bras Pneumol. 2008;34(9):702-6.
12. Hillman DR, Finucane KE. A model of the respiratory pump. J Appl Physiol. 1987;63(3):951-61.
13. Wilson TA. Mechanics of compartmental models of the chest wall. J Appl Physiol. 1988;65(5):2261-4.
14. Faithfull D, Jones JG, Jordan C. Measurement of the relative contributions of rib cage and abdomen/diaphragm to tidal breathing in man. Br J Anaesth. 1979;51(5):391-8.
15. Tobin MJ. Breathing pattern analysis. Intensive Care Med. 1992;18(4):193-201.
16. Hyatt RE, Schilder DP, Fry DL. Relationship between maximum expiratory flow and degree of lung inflation. J Appl Physiol. 1958;13(3):331-6.
17. Hyatt RE, Wilcox RE. The pressure-flow relationships of the intrathoracic airway in man. J Clin Invest. 1963;42:29-39.
18. Russell RI, Helms PJ. Evaluation of three different techniques used to measure chest wall movements in children. Eur Respir J. 1994;7(11):2073-6.
19. Masa JF, Corral J, Martín MJ, Riesco JA, Sojo A, Hernández M, et al. Assessment of thoracoabdominal bands to detect respiratory effort-related arousal. Eur Respir J. 2003;22(4):661-7.
20. Sanna A, Bertoli F, Misuri G, Gigliotti F, Iandelli I, Mancini M, et al. Chest wall kinematics and respiratory muscle action in walking healthy humans. J Appl Physiol. 1999;87(3):938-46.
21. Peacock AJ, Morgan MD, Gourlay S, Turton C, Denison DM. Optical mapping of the thoracoabdominal wall. Thorax. 1984;39(2):93-100.
22. Paulin E, Brunetto AF, Carvalho CR. Efeitos de programa de exercícios físicos direcionado ao aumento da mobilidade torácica em pacientes portadores de doença pulmonar obstrutiva crônica. J Pneumol. 2003;29(5):287-94.
23. Kunikoshita LN, Silva YP, Silva TL, Costa D, Jamami M. Efeitos de três programas de Fisioterapia Respiratória (PFR) em portadores de DPOC. Rev Bras Fisioter. 2006;10(4):449-55.
24. Moreno MA, Catai AM, Teodori RM, Borges BL, Cesar MC, Silva E. Effect of a muscle stretching program using the Global Postural Reeducation method on respiratory muscle strength and thoracoabdominal mobility of sedentary young males. J Bras Pneumol. 2007;33(6):679-86.
25. Hawkins P, Davison AG, Dasgupta B, Moxham J. Diaphragm strength in acute systemic lupus erythematosus in a patient with paradoxical abdominal motion and reduced lung volumes. Thorax. 2001;56(4):329-30.
26. Slader CA, Reddel HK, Spencer LM, Belousova EG, Armour CL, Bosnic-Anticevich SZ, et al. Double blind randomised controlled trial of two different breathing techniques in the management of asthma. Thorax. 2006;61(8):651-6.
About the authors
Denise da Vinha Ricieri
Assistant Professor in the School of Physical Therapy. Universidade Federal do Paraná - UFRP, Federal University of Paraná - Curitiba, Brazil.
Nelson Augusto Rosário Filho
Full Professor in the Pediatrics Department. Universidade Federal do Paraná - UFPR, Federal University of Paraná - Curitiba, Brazil.
Study carried out under the auspices of the Postgraduate Program in Child and Adolescent Health of the Pediatrics Department. Universidade Federal do Paraná - UFPR, Federal University of Paraná - Curitiba, Brazil.
Correspondence to: Denise da Vinha Ricieri. Hospital de Clínicas da UFPR, Pronto-atendimento Pediátrico, Serviço de Alergia, Imunologia e Pneumologia Pediátrica, Rua General Carneiro, 181, CEP 80060-900, Curitiba, PR, Brasil.
Tel 55 41 3452 2919. E-mail: denise.ricieri@ufpr.br
Financial support: None.
Submitted: 29 May 2008. Accepted, after review: 07 July 2008.